Initiation factor eIF2-independent mode of c-Src mRNA translation occurs via an internal ribosome entry site
- PMID: 20028973
- PMCID: PMC2820799
- DOI: 10.1074/jbc.M109.029462
Initiation factor eIF2-independent mode of c-Src mRNA translation occurs via an internal ribosome entry site
Abstract
Overexpression and activation of the c-Src protein have been linked to the development of a wide variety of cancers. The molecular mechanism(s) of c-Src overexpression in cancer cells is not clear. We report here an internal ribosome entry site (IRES) in the c-Src mRNA that is constituted by both 5'-noncoding and -coding regions. The inhibition of cap-dependent translation by m(7)GDP in the cell-free translation system or induction of endoplasmic reticulum stress in hepatoma-derived cells resulted in stimulation of the c-Src IRES activities. Sucrose density gradient analyses revealed formation of a stable binary complex between the c-Src IRES and purified HeLa 40 S ribosomal subunit in the absence of initiation factors. We further demonstrate eIF2-independent assembly of 80 S initiation complex on the c-Src IRES. These features of the c-Src IRES appear to be reminiscent of that of hepatitis C virus-like IRESs and translation initiation in prokaryotes. Transfection studies and genetic analysis revealed that the c-Src IRES permitted initiation at the authentic AUG351, which is also used for conventional translation initiation of the c-Src mRNA. Our studies unveiled a novel regulatory mechanism of c-Src synthesis mediated by an IRES element, which exhibits enhanced activity during cellular stress and is likely to cause c-Src overexpression during oncogenesis and metastasis.
Figures
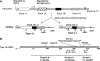
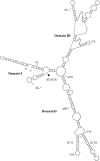
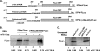
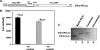

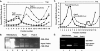
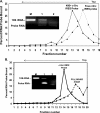
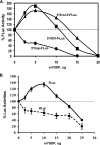
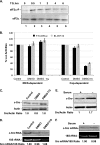
Similar articles
-
Hepatitis C Virus Translation Regulation.Int J Mol Sci. 2020 Mar 27;21(7):2328. doi: 10.3390/ijms21072328. Int J Mol Sci. 2020. PMID: 32230899 Free PMC article. Review.
-
Canonical initiation factor requirements of the Myc family of internal ribosome entry segments.Mol Cell Biol. 2009 Mar;29(6):1565-74. doi: 10.1128/MCB.01283-08. Epub 2009 Jan 5. Mol Cell Biol. 2009. PMID: 19124605 Free PMC article.
-
Eukaryotic translation initiation factor 4GI and p97 promote cellular internal ribosome entry sequence-driven translation.Proc Natl Acad Sci U S A. 2005 Sep 20;102(38):13421-6. doi: 10.1073/pnas.0506536102. Epub 2005 Sep 7. Proc Natl Acad Sci U S A. 2005. PMID: 16174738 Free PMC article.
-
Translation-competent 48S complex formation on HCV IRES requires the RNA-binding protein NSAP1.Nucleic Acids Res. 2011 Sep 1;39(17):7791-802. doi: 10.1093/nar/gkr509. Epub 2011 Jun 28. Nucleic Acids Res. 2011. PMID: 21715376 Free PMC article.
-
More than just scanning: the importance of cap-independent mRNA translation initiation for cellular stress response and cancer.Cell Mol Life Sci. 2017 May;74(9):1659-1680. doi: 10.1007/s00018-016-2428-2. Epub 2016 Dec 2. Cell Mol Life Sci. 2017. PMID: 27913822 Free PMC article. Review.
Cited by
-
eIF3j inhibits translation of a subset of circular RNAs in eukaryotic cells.Nucleic Acids Res. 2022 Nov 11;50(20):11529-11549. doi: 10.1093/nar/gkac980. Nucleic Acids Res. 2022. PMID: 36330957 Free PMC article.
-
An early nonsense mutation facilitates the expression of a short isoform of CNGA3 by alternative translation initiation.Exp Eye Res. 2018 Jun;171:48-53. doi: 10.1016/j.exer.2018.02.027. Epub 2018 Feb 27. Exp Eye Res. 2018. PMID: 29499183 Free PMC article.
-
The structures of nonprotein-coding RNAs that drive internal ribosome entry site function.Wiley Interdiscip Rev RNA. 2012 Mar-Apr;3(2):195-212. doi: 10.1002/wrna.1105. Epub 2012 Jan 3. Wiley Interdiscip Rev RNA. 2012. PMID: 22215521 Free PMC article. Review.
-
Ectopic pregnancy-derived human trophoblastic stem cells regenerate dopaminergic nigrostriatal pathway to treat parkinsonian rats.PLoS One. 2012;7(12):e52491. doi: 10.1371/journal.pone.0052491. Epub 2012 Dec 21. PLoS One. 2012. Retraction in: PLoS One. 2021 Feb 11;16(2):e0247151. doi: 10.1371/journal.pone.0247151 PMID: 23285066 Free PMC article. Retracted.
-
Hepatitis C virus-induced cancer stem cell-like signatures in cell culture and murine tumor xenografts.J Virol. 2011 Dec;85(23):12292-303. doi: 10.1128/JVI.05920-11. Epub 2011 Sep 21. J Virol. 2011. PMID: 21937640 Free PMC article.
References
Publication types
MeSH terms
Substances
Grants and funding
LinkOut - more resources
Full Text Sources
Miscellaneous