Ethylene interacts with abscisic acid to regulate endosperm rupture during germination: a comparative approach using Lepidium sativum and Arabidopsis thaliana
- PMID: 20023197
- PMCID: PMC2814513
- DOI: 10.1105/tpc.109.070201
Ethylene interacts with abscisic acid to regulate endosperm rupture during germination: a comparative approach using Lepidium sativum and Arabidopsis thaliana
Abstract
The micropylar endosperm cap covering the radicle in the mature seeds of most angiosperms acts as a constraint that regulates seed germination. Here, we report on a comparative seed biology study with the close Brassicaceae relatives Lepidium sativum and Arabidopsis thaliana showing that ethylene biosynthesis and signaling regulate seed germination by a mechanism that requires the coordinated action of the radicle and the endosperm cap. The larger seed size of Lepidium allows direct tissue-specific biomechanical, biochemical, and transcriptome analyses. We show that ethylene promotes endosperm cap weakening of Lepidium and endosperm rupture of both species and that it counteracts the inhibitory action of abscisic acid (ABA) on these two processes. Cross-species microarrays of the Lepidium micropylar endosperm cap and the radicle show that the ethylene-ABA antagonism involves both tissues and has the micropylar endosperm cap as a major target. Ethylene counteracts the ABA-induced inhibition without affecting seed ABA levels. The Arabidopsis loss-of-function mutants ACC oxidase2 (aco2; ethylene biosynthesis) and constitutive triple response1 (ethylene signaling) are impaired in the 1-aminocyclopropane-1-carboxylic acid (ACC)-mediated reversion of the ABA-induced inhibition of seed germination. Ethylene production by the ACC oxidase orthologs Lepidium ACO2 and Arabidopsis ACO2 appears to be a key regulatory step. Endosperm cap weakening and rupture are promoted by ethylene and inhibited by ABA to regulate germination in a process conserved across the Brassicaceae.
Figures
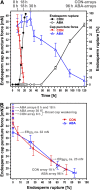
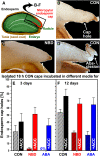
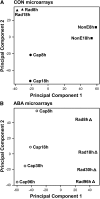
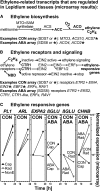
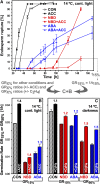
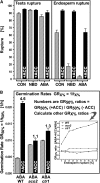
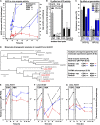
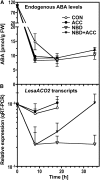
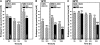
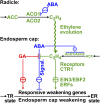
Similar articles
-
Endosperm-limited Brassicaceae seed germination: abscisic acid inhibits embryo-induced endosperm weakening of Lepidium sativum (cress) and endosperm rupture of cress and Arabidopsis thaliana.Plant Cell Physiol. 2006 Jul;47(7):864-77. doi: 10.1093/pcp/pcj059. Epub 2006 May 16. Plant Cell Physiol. 2006. PMID: 16705010
-
Regulation of seed germination in the close Arabidopsis relative Lepidium sativum: a global tissue-specific transcript analysis.Plant Physiol. 2011 Apr;155(4):1851-70. doi: 10.1104/pp.110.169706. Epub 2011 Feb 14. Plant Physiol. 2011. PMID: 21321254 Free PMC article.
-
Cross-species approaches to seed dormancy and germination: conservation and biodiversity of ABA-regulated mechanisms and the Brassicaceae DOG1 genes.Plant Mol Biol. 2010 May;73(1-2):67-87. doi: 10.1007/s11103-009-9583-x. Epub 2009 Dec 15. Plant Mol Biol. 2010. PMID: 20013031
-
Beyond gibberellins and abscisic acid: how ethylene and jasmonates control seed germination.Plant Cell Rep. 2012 Feb;31(2):253-70. doi: 10.1007/s00299-011-1180-1. Epub 2011 Nov 2. Plant Cell Rep. 2012. PMID: 22044964 Review.
-
Underlying Biochemical and Molecular Mechanisms for Seed Germination.Int J Mol Sci. 2022 Jul 31;23(15):8502. doi: 10.3390/ijms23158502. Int J Mol Sci. 2022. PMID: 35955637 Free PMC article. Review.
Cited by
-
A tale of two tissues: AtGH9C1 is an endo-β-1,4-glucanase involved in root hair and endosperm development in Arabidopsis.PLoS One. 2012;7(11):e49363. doi: 10.1371/journal.pone.0049363. Epub 2012 Nov 16. PLoS One. 2012. PMID: 23173056 Free PMC article.
-
Global Transcriptomic Analysis Reveals the Mechanism of Phelipanche aegyptiaca Seed Germination.Int J Mol Sci. 2016 Jul 15;17(7):1139. doi: 10.3390/ijms17071139. Int J Mol Sci. 2016. PMID: 27428962 Free PMC article.
-
Effects of abscisic acid, ethylene and sugars on the mobilization of storage proteins and carbohydrates in seeds of the tropical tree Sesbania virgata (Leguminosae).Ann Bot. 2010 Oct;106(4):607-16. doi: 10.1093/aob/mcq159. Epub 2010 Aug 12. Ann Bot. 2010. PMID: 20705626 Free PMC article.
-
Label-Free Quantitative Proteomics Reveal the Involvement of PRT6 in Arabidopsis thaliana Seed Responsiveness to Ethylene.Int J Mol Sci. 2022 Aug 19;23(16):9352. doi: 10.3390/ijms23169352. Int J Mol Sci. 2022. PMID: 36012613 Free PMC article.
-
A wheat homolog of MOTHER OF FT AND TFL1 acts in the regulation of germination.Plant Cell. 2011 Sep;23(9):3215-29. doi: 10.1105/tpc.111.088492. Epub 2011 Sep 6. Plant Cell. 2011. PMID: 21896881 Free PMC article.
References
-
- Alonso, J.M., and Ecker, J.R. (2001). The ethylene pathway: A paradigm for plant hormone signaling and interaction. Sci. STKE 2001 RE1. - PubMed
-
- Bar-Or, C., Czosnek, H., and Koltai, H. (2007). Cross-species microarray hybridizations: A developing tool for studying species diversity. Trends Genet. 23 200–207. - PubMed
Publication types
MeSH terms
Substances
Grants and funding
LinkOut - more resources
Full Text Sources
Molecular Biology Databases
Miscellaneous