A rotavirus spike protein conformational intermediate binds lipid bilayers
- PMID: 20007281
- PMCID: PMC2812363
- DOI: 10.1128/JVI.01682-09
A rotavirus spike protein conformational intermediate binds lipid bilayers
Abstract
During rotavirus entry, a virion penetrates a host cell membrane, sheds its outer capsid proteins, and releases a transcriptionally active subviral particle into the cytoplasm. VP5, the rotavirus protein believed to interact with the membrane bilayer, is a tryptic cleavage product of the outer capsid spike protein, VP4. When a rotavirus particle uncoats, VP5 folds back, in a rearrangement that resembles the fusogenic conformational changes in enveloped-virus fusion proteins. We present direct experimental evidence that this rearrangement leads to membrane binding. VP5 does not associate with liposomes when mounted as part of the trypsin-primed spikes on intact virions, nor does it do so after it has folded back into a stably trimeric, low-energy state. But it does bind liposomes when they are added to virions before uncoating, and VP5 rearrangement is then triggered by addition of EDTA. The presence of liposomes during the rearrangement enhances the otherwise inefficient VP5 conformational change. A VP5 fragment, VP5CT, produced from monomeric recombinant VP4 by successive treatments with chymotrypsin and trypsin, also binds liposomes only when the proteolysis proceeds in their presence. A monoclonal antibody that neutralizes infectivity by blocking a postattachment entry event also blocks VP5 liposome association. We propose that VP5 binds lipid bilayers in an intermediate conformational state, analogous to the extended intermediate conformation of enveloped-virus fusion proteins.
Figures
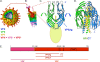
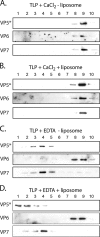
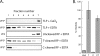
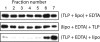
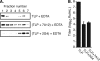
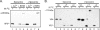
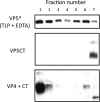
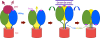
Similar articles
-
VP5* rearranges when rotavirus uncoats.J Virol. 2009 Nov;83(21):11372-7. doi: 10.1128/JVI.01228-09. Epub 2009 Aug 19. J Virol. 2009. PMID: 19692464 Free PMC article.
-
Effect of mutations in VP5 hydrophobic loops on rotavirus cell entry.J Virol. 2010 Jun;84(12):6200-7. doi: 10.1128/JVI.02461-09. Epub 2010 Apr 7. J Virol. 2010. PMID: 20375171 Free PMC article.
-
Proteolysis of monomeric recombinant rotavirus VP4 yields an oligomeric VP5* core.J Virol. 2001 Aug;75(16):7339-50. doi: 10.1128/JVI.75.16.7339-7350.2001. J Virol. 2001. PMID: 11462006 Free PMC article.
-
Emerging themes in rotavirus cell entry, genome organization, transcription and replication.Virus Res. 2004 Apr;101(1):67-81. doi: 10.1016/j.virusres.2003.12.007. Virus Res. 2004. PMID: 15010218 Review.
-
Rotavirus Replication: Gaps of Knowledge on Virus Entry and Morphogenesis.Tohoku J Exp Med. 2019 Aug;248(4):285-296. doi: 10.1620/tjem.248.285. Tohoku J Exp Med. 2019. PMID: 31447474 Review.
Cited by
-
Interactions among capsid proteins orchestrate rotavirus particle functions.Curr Opin Virol. 2012 Aug;2(4):373-9. doi: 10.1016/j.coviro.2012.04.005. Epub 2012 May 16. Curr Opin Virol. 2012. PMID: 22595300 Free PMC article. Review.
-
ERdj5 Reductase Cooperates with Protein Disulfide Isomerase To Promote Simian Virus 40 Endoplasmic Reticulum Membrane Translocation.J Virol. 2015 Sep;89(17):8897-908. doi: 10.1128/JVI.00941-15. Epub 2015 Jun 17. J Virol. 2015. PMID: 26085143 Free PMC article.
-
Glycosphingolipids as receptors for non-enveloped viruses.Viruses. 2010 Apr;2(4):1011-1049. doi: 10.3390/v2041011. Epub 2010 Apr 15. Viruses. 2010. PMID: 21994669 Free PMC article.
-
Rhesus rotavirus trafficking during entry into MA104 cells is restricted to the early endosome compartment.J Virol. 2012 Apr;86(7):4009-13. doi: 10.1128/JVI.06667-11. Epub 2012 Jan 25. J Virol. 2012. PMID: 22278225 Free PMC article.
-
Single-Particle Detection of Transcription following Rotavirus Entry.J Virol. 2017 Aug 24;91(18):e00651-17. doi: 10.1128/JVI.00651-17. Print 2017 Sep 15. J Virol. 2017. PMID: 28701394 Free PMC article.
References
-
- Ciarlet, M., J. E. Ludert, M. Iturriza-Gomara, F. Liprandi, J. J. Gray, U. Desselberger, and M. K. Estes. 2002. The initial interaction of rotavirus strains with N-acetyl-neuraminic (sialic) acid residues on the cell surface correlates with VP4 genotype, not species of origin. J. Virol. 76:4087-4095. - PMC - PubMed
Publication types
MeSH terms
Substances
Grants and funding
LinkOut - more resources
Full Text Sources
Other Literature Sources