Severe osteogenesis imperfecta in cyclophilin B-deficient mice
- PMID: 19997487
- PMCID: PMC2777385
- DOI: 10.1371/journal.pgen.1000750
Severe osteogenesis imperfecta in cyclophilin B-deficient mice
Abstract
Osteogenesis Imperfecta (OI) is a human syndrome characterized by exquisitely fragile bones due to osteoporosis. The majority of autosomal dominant OI cases result from point or splice site mutations in the type I collagen genes, which are thought to lead to aberrant osteoid within developing bones. OI also occurs in humans with homozygous mutations in Prolyl-3-Hydroxylase-1 (LEPRE1). Although P3H1 is known to hydroxylate a single residue (pro-986) in type I collagen chains, it is unclear how this modification acts to facilitate collagen fibril formation. P3H1 exists in a complex with CRTAP and the peptidyl-prolyl isomerase cyclophilin B (CypB), encoded by the Ppib gene. Mutations in CRTAP cause OI in mice and humans, through an unknown mechanism, while the role of CypB in this complex has been a complete mystery. To study the role of mammalian CypB, we generated mice lacking this protein. Early in life, Ppib-/- mice developed kyphosis and severe osteoporosis. Collagen fibrils in Ppib-/- mice had abnormal morphology, further consistent with an OI phenotype. In vitro studies revealed that in CypB-deficient fibroblasts, procollagen did not localize properly to the golgi. We found that levels of P3H1 were substantially reduced in Ppib-/- cells, while CRTAP was unaffected by loss of CypB. Conversely, knockdown of either P3H1 or CRTAP did not affect cellular levels of CypB, but prevented its interaction with collagen in vitro. Furthermore, knockdown of CRTAP also caused depletion of cellular P3H1. Consistent with these changes, post translational prolyl-3-hydroxylation of type I collagen by P3H1 was essentially absent in CypB-deficient cells and tissues from CypB-knockout mice. These data provide significant new mechanistic insight into the pathophysiology of OI and reveal how the members of the P3H1/CRTAP/CypB complex interact to direct proper formation of collagen and bone.
Conflict of interest statement
The authors have declared that no competing interests exist.
Figures
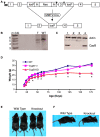
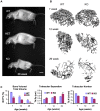
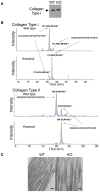
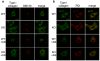
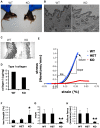
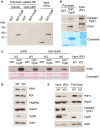
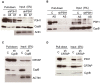
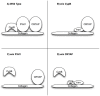
Similar articles
-
Mutations in PPIB (cyclophilin B) delay type I procollagen chain association and result in perinatal lethal to moderate osteogenesis imperfecta phenotypes.Hum Mol Genet. 2011 Apr 15;20(8):1595-609. doi: 10.1093/hmg/ddr037. Epub 2011 Jan 31. Hum Mol Genet. 2011. PMID: 21282188 Free PMC article.
-
Prolyl 3-hydroxylase 1 and CRTAP are mutually stabilizing in the endoplasmic reticulum collagen prolyl 3-hydroxylation complex.Hum Mol Genet. 2010 Jan 15;19(2):223-34. doi: 10.1093/hmg/ddp481. Epub 2009 Oct 21. Hum Mol Genet. 2010. PMID: 19846465 Free PMC article.
-
PPIB mutations cause severe osteogenesis imperfecta.Am J Hum Genet. 2009 Oct;85(4):521-7. doi: 10.1016/j.ajhg.2009.09.001. Epub 2009 Sep 24. Am J Hum Genet. 2009. PMID: 19781681 Free PMC article.
-
Null mutations in LEPRE1 and CRTAP cause severe recessive osteogenesis imperfecta.Cell Tissue Res. 2010 Jan;339(1):59-70. doi: 10.1007/s00441-009-0872-0. Epub 2009 Oct 28. Cell Tissue Res. 2010. PMID: 19862557 Free PMC article. Review.
-
Components of the collagen prolyl 3-hydroxylation complex are crucial for normal bone development.Cell Cycle. 2007 Jul 15;6(14):1675-81. doi: 10.4161/cc.6.14.4474. Epub 2007 May 18. Cell Cycle. 2007. PMID: 17630507 Review.
Cited by
-
Generalized connective tissue disease in Crtap-/- mouse.PLoS One. 2010 May 11;5(5):e10560. doi: 10.1371/journal.pone.0010560. PLoS One. 2010. PMID: 20485499 Free PMC article.
-
Cyclophilin B supports Myc and mutant p53-dependent survival of glioblastoma multiforme cells.Cancer Res. 2014 Jan 15;74(2):484-96. doi: 10.1158/0008-5472.CAN-13-0771. Epub 2013 Nov 22. Cancer Res. 2014. PMID: 24272483 Free PMC article.
-
Extracellular cyclophilins in health and disease.Biochim Biophys Acta. 2015 Oct;1850(10):2087-95. doi: 10.1016/j.bbagen.2014.11.013. Epub 2014 Nov 18. Biochim Biophys Acta. 2015. PMID: 25445705 Free PMC article. Review.
-
Cyclosporin A impairs the secretion and activity of ADAMTS13 (a disintegrin and metalloprotease with thrombospondin type 1 repeat).J Biol Chem. 2012 Dec 28;287(53):44361-71. doi: 10.1074/jbc.M112.383968. Epub 2012 Nov 9. J Biol Chem. 2012. PMID: 23144461 Free PMC article.
-
Differential effects of collagen prolyl 3-hydroxylation on skeletal tissues.PLoS Genet. 2014 Jan;10(1):e1004121. doi: 10.1371/journal.pgen.1004121. Epub 2014 Jan 23. PLoS Genet. 2014. PMID: 24465224 Free PMC article.
References
-
- Martin E, Shapiro JR. Osteogenesis imperfecta:epidemiology and pathophysiology. Curr Osteoporos Rep. 2007;5:91–97. - PubMed
-
- Byers PH. New York: Wiley-Liss; 1993. Osteogenesis imperfecta. pp. 317–350.
-
- Morello R, Bertin TK, Chen Y, Hicks J, Tonachini L, et al. CRTAP is required for prolyl 3- hydroxylation and mutations cause recessive osteogenesis imperfecta. Cell. 2006;127:291–304. - PubMed
Publication types
MeSH terms
Substances
LinkOut - more resources
Full Text Sources
Other Literature Sources
Medical
Molecular Biology Databases