Structure and hydration of membranes embedded with voltage-sensing domains
- PMID: 19940918
- PMCID: PMC2784928
- DOI: 10.1038/nature08542
Structure and hydration of membranes embedded with voltage-sensing domains
Abstract
Despite the growing number of atomic-resolution membrane protein structures, direct structural information about proteins in their native membrane environment is scarce. This problem is particularly relevant in the case of the highly charged S1-S4 voltage-sensing domains responsible for nerve impulses, where interactions with the lipid bilayer are critical for the function of voltage-activated ion channels. Here we use neutron diffraction, solid-state nuclear magnetic resonance (NMR) spectroscopy and molecular dynamics simulations to investigate the structure and hydration of bilayer membranes containing S1-S4 voltage-sensing domains. Our results show that voltage sensors adopt transmembrane orientations and cause a modest reshaping of the surrounding lipid bilayer, and that water molecules intimately interact with the protein within the membrane. These structural findings indicate that voltage sensors have evolved to interact with the lipid membrane while keeping energetic and structural perturbations to a minimum, and that water penetrates the membrane, to hydrate charged residues and shape the transmembrane electric field.
Figures
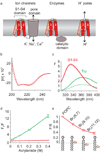
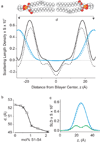
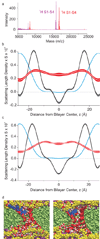
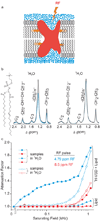
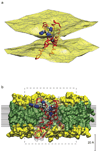
Comment in
-
Structural biology: Highly charged meetings.Nature. 2009 Nov 26;462(7272):420-1. doi: 10.1038/462420a. Nature. 2009. PMID: 19940907 No abstract available.
Similar articles
-
Structural interactions between lipids, water and S1-S4 voltage-sensing domains.J Mol Biol. 2012 Nov 2;423(4):632-47. doi: 10.1016/j.jmb.2012.07.015. Epub 2012 Jul 31. J Mol Biol. 2012. PMID: 22858867 Free PMC article.
-
How does a voltage sensor interact with a lipid bilayer? Simulations of a potassium channel domain.Structure. 2007 Feb;15(2):235-44. doi: 10.1016/j.str.2007.01.004. Structure. 2007. PMID: 17292841 Free PMC article.
-
Structural dynamics of the S4 voltage-sensor helix in lipid bilayers lacking phosphate groups.J Phys Chem B. 2011 Jul 14;115(27):8732-8. doi: 10.1021/jp2001964. Epub 2011 Jun 22. J Phys Chem B. 2011. PMID: 21692541 Free PMC article.
-
The voltage-sensor structure in a voltage-gated channel.Trends Biochem Sci. 2005 Apr;30(4):166-8. doi: 10.1016/j.tibs.2005.02.006. Trends Biochem Sci. 2005. PMID: 15817390 Review.
-
Sensing voltage across lipid membranes.Nature. 2008 Dec 18;456(7224):891-7. doi: 10.1038/nature07620. Nature. 2008. PMID: 19092925 Free PMC article. Review.
Cited by
-
Microscopic origin of gating current fluctuations in a potassium channel voltage sensor.Biophys J. 2012 Jun 6;102(11):L44-6. doi: 10.1016/j.bpj.2012.04.021. Epub 2012 Jun 5. Biophys J. 2012. PMID: 22713585 Free PMC article.
-
Structure of the disulfide bond generating membrane protein DsbB in the lipid bilayer.J Mol Biol. 2013 May 27;425(10):1670-82. doi: 10.1016/j.jmb.2013.02.009. Epub 2013 Feb 14. J Mol Biol. 2013. PMID: 23416557 Free PMC article.
-
Computer Simulations of Voltage-Gated Cation Channels.J Phys Chem Lett. 2012 Mar 29;3:1017-1023. doi: 10.1021/jz300089g. J Phys Chem Lett. 2012. PMID: 22523619 Free PMC article.
-
Characterization of the water defect at the HIV-1 gp41 membrane spanning domain in bilayers with and without cholesterol using molecular simulations.Biochim Biophys Acta. 2014 May;1838(5):1396-405. doi: 10.1016/j.bbamem.2014.01.009. Epub 2014 Jan 16. Biochim Biophys Acta. 2014. PMID: 24440660 Free PMC article.
-
Spontaneous membrane-translocating peptides by orthogonal high-throughput screening.J Am Chem Soc. 2011 Jun 15;133(23):8995-9004. doi: 10.1021/ja2017416. Epub 2011 May 19. J Am Chem Soc. 2011. PMID: 21545169 Free PMC article.
References
-
- Murata Y, Iwasaki H, Sasaki M, Inaba K, Okamura Y. Phosphoinositide phosphatase activity coupled to an intrinsic voltage sensor. Nature. 2005;435:1239–1243. - PubMed
-
- Jiang Y, et al. X-ray structure of a voltage-dependent K+ channel. Nature. 2003;423:33–41. - PubMed
-
- Long SB, Tao X, Campbell EB, MacKinnon R. Atomic structure of a voltage-dependent K+ channel in a lipid membrane-like environment. Nature. 2007;450:376–382. - PubMed