Dominant negative mutant actins identified in flightless Drosophila can be classified into three classes
- PMID: 19933578
- PMCID: PMC2836038
- DOI: 10.1074/jbc.M109.059881
Dominant negative mutant actins identified in flightless Drosophila can be classified into three classes
Abstract
Strongly dominant negative mutant actins, identified by An and Mogami (An, H. S., and Mogami, K. (1996) J. Mol. Biol. 260, 492-505), in the indirect flight muscle of Drosophila impaired its flight, even when three copies of the wild-type gene were present. Understanding how these strongly dominant negative mutant actins disrupt the function of wild-type actin would provide useful information about the molecular mechanism by which actin functions in vivo. Here, we expressed and purified six of these strongly dominant negative mutant actins in Dictyostelium and classified them into three groups based on their biochemical phenotypes. The first group, G156D, G156S, and G268D actins, showed impaired polymerization and a tendency to aggregate under conditions favoring polymerization. G63D actin of the second group was also unable to polymerize but, unlike those in the first group, remained soluble under polymerizing conditions. Kinetic analyses using G63D actin or G63D actin.gelsolin complexes suggested that the pointed end surface is defective, which would alter the polymerization kinetics of wild-type actin when mixed and could affect formation of thin filament structures in indirect flight muscle. The third group, R95C and E226K actins, was normal in terms of polymerization, but their motility on heavy meromyosin surfaces in the presence of tropomyosin-troponin indicated altered sensitivity to Ca(2+). Cofilaments in which R95C or E226K actins were copolymerized with a 3-fold excess of wild-type actin also showed altered Ca(2+) sensitivity in the presence of tropomyosin-troponin.
Figures
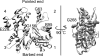
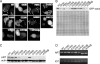
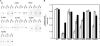
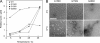
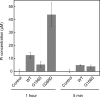
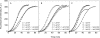
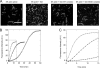
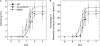
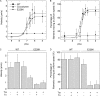
Similar articles
-
Structural basis for the higher Ca(2+)-activation of the regulated actin-activated myosin ATPase observed with Dictyostelium/Tetrahymena actin chimeras.J Mol Biol. 2000 Feb 18;296(2):579-95. doi: 10.1006/jmbi.1999.3467. J Mol Biol. 2000. PMID: 10669610
-
A novel system for expressing toxic actin mutants in Dictyostelium and purification and characterization of a dominant lethal yeast actin mutant.J Biol Chem. 2007 Sep 21;282(38):27721-7. doi: 10.1074/jbc.M703165200. Epub 2007 Jul 26. J Biol Chem. 2007. PMID: 17656358
-
Mini-thin filaments regulated by troponin-tropomyosin.Proc Natl Acad Sci U S A. 2005 Jan 18;102(3):656-61. doi: 10.1073/pnas.0407225102. Epub 2005 Jan 11. Proc Natl Acad Sci U S A. 2005. PMID: 15644437 Free PMC article.
-
Tropomyosin-binding site(s) on the Dictyostelium actin surface as identified by site-directed mutagenesis.Biochemistry. 1996 Nov 19;35(46):14465-72. doi: 10.1021/bi961292c. Biochemistry. 1996. PMID: 8931542
-
Caldesmon and thin-filament regulation of muscle contraction.Cell Biophys. 1988 Jan-Jun;12:73-85. doi: 10.1007/BF02918351. Cell Biophys. 1988. PMID: 2453287 Review.
Cited by
-
Live-cell imaging of actin dynamics reveals mechanisms of stereocilia length regulation in the inner ear.Nat Commun. 2015 Apr 21;6:6873. doi: 10.1038/ncomms7873. Nat Commun. 2015. PMID: 25898120 Free PMC article.
-
A periodic pattern of evolutionarily conserved basic and acidic residues constitutes the binding interface of actin-tropomyosin.J Biol Chem. 2013 Apr 5;288(14):9602-9609. doi: 10.1074/jbc.M113.451161. Epub 2013 Feb 18. J Biol Chem. 2013. PMID: 23420843 Free PMC article.
-
Rapid nucleotide exchange renders Asp-11 mutant actins resistant to depolymerizing activity of cofilin, leading to dominant toxicity in vivo.J Biol Chem. 2013 Jan 18;288(3):1739-49. doi: 10.1074/jbc.M112.404657. Epub 2012 Dec 3. J Biol Chem. 2013. PMID: 23212920 Free PMC article.
-
K336I mutant actin alters the structure of neighbouring protomers in filaments and reduces affinity for actin-binding proteins.Sci Rep. 2019 Mar 29;9(1):5353. doi: 10.1038/s41598-019-41795-w. Sci Rep. 2019. PMID: 30926871 Free PMC article.
References
-
- Pollard T. D., Blanchoin L., Mullins R. D. (2000) Annu. Rev. Biophys. Biomol. Struct. 29, 545–576 - PubMed
-
- Carlier M. F. (1992) Philos. Trans. R. Soc. Lond. B Biol. Sci. 336, 93–97 - PubMed
-
- Schüler H. (2001) Biochim. Biophys. Acta 1549, 137–147 - PubMed
-
- Prochniewicz E., Yanagida T. (1990) J. Mol. Biol. 216, 761–772 - PubMed
Publication types
MeSH terms
Substances
LinkOut - more resources
Full Text Sources
Molecular Biology Databases
Research Materials
Miscellaneous