Correlations of neuronal and microvascular densities in murine cortex revealed by direct counting and colocalization of nuclei and vessels
- PMID: 19923289
- PMCID: PMC4972024
- DOI: 10.1523/JNEUROSCI.3287-09.2009
Correlations of neuronal and microvascular densities in murine cortex revealed by direct counting and colocalization of nuclei and vessels
Abstract
It is well known that the density of neurons varies within the adult brain. In neocortex, this includes variations in neuronal density between different lamina as well as between different regions. Yet the concomitant variation of the microvessels is largely uncharted. Here, we present automated histological, imaging, and analysis tools to simultaneously map the locations of all neuronal and non-neuronal nuclei and the centerlines and diameters of all blood vessels within thick slabs of neocortex from mice. Based on total inventory measurements of different cortical regions ( approximately 10(7) cells vectorized across brains), these methods revealed: (1) In three dimensions, the mean distance of the center of neuronal somata to the closest microvessel was 15 mum. (2) Volume samples within lamina of a given region show that the density of microvessels does not match the strong laminar variation in neuronal density. This holds for both agranular and granular cortex. (3) Volume samples in successive radii from the midline to the ventral-lateral edge, where each volume summed the number of cells and microvessels from the pia to the white matter, show a significant correlation between neuronal and microvessel densities. These data show that while neuronal and vascular densities do not track each other on the 100 mum scale of cortical lamina, they do track each other on the 1-10 mm scale of the cortical mantle. The absence of a disproportionate density of blood vessels in granular lamina is argued to be consistent with the initial locus of functional brain imaging signals.
Figures
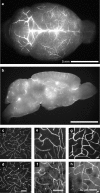
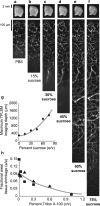
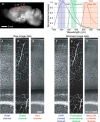
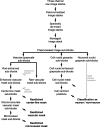
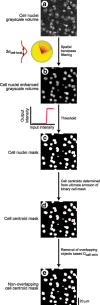
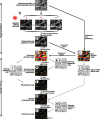
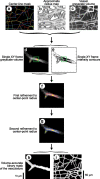
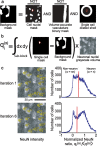
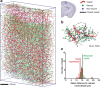
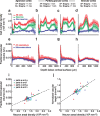
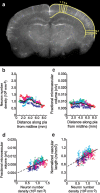
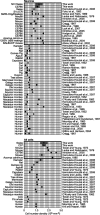
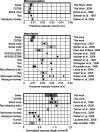
Similar articles
-
Numerical and length densities of microvessels in the human brain: Correlation with preferential orientation of microvessels in the cerebral cortex, subcortical grey matter and white matter, pons and cerebellum.J Chem Neuroanat. 2018 Mar;88:22-32. doi: 10.1016/j.jchemneu.2017.11.005. Epub 2017 Nov 4. J Chem Neuroanat. 2018. PMID: 29113946
-
GABA neurons provide a rich input to microvessels but not nitric oxide neurons in the rat cerebral cortex: a means for direct regulation of local cerebral blood flow.J Comp Neurol. 2000 May 29;421(2):161-71. J Comp Neurol. 2000. PMID: 10813779
-
Quantification of neuronal density across cortical depth using automated 3D analysis of confocal image stacks.Brain Struct Funct. 2017 Sep;222(7):3333-3353. doi: 10.1007/s00429-017-1382-6. Epub 2017 Feb 27. Brain Struct Funct. 2017. PMID: 28243763 Free PMC article.
-
Neuronal units linked to microvascular modules in cerebral cortex: response elements for imaging the brain.Cereb Cortex. 1996 Sep-Oct;6(5):647-60. doi: 10.1093/cercor/6.5.647. Cereb Cortex. 1996. PMID: 8921201 Review.
-
Scaling of cortical neuron density and white matter volume in mammals.J Hirnforsch. 1997;38(4):513-24. J Hirnforsch. 1997. PMID: 9476216 Review.
Cited by
-
Cell lines and clearing approaches: a single-cell level 3D light-sheet fluorescence microscopy dataset of multicellular spheroids.Data Brief. 2021 Apr 23;36:107090. doi: 10.1016/j.dib.2021.107090. eCollection 2021 Jun. Data Brief. 2021. PMID: 34026984 Free PMC article.
-
Staining and embedding the whole mouse brain for electron microscopy.Nat Methods. 2012 Dec;9(12):1198-201. doi: 10.1038/nmeth.2213. Epub 2012 Oct 21. Nat Methods. 2012. PMID: 23085613
-
A molecular mechanism explaining albuminuria in kidney disease.Nat Metab. 2020 May;2(5):461-474. doi: 10.1038/s42255-020-0204-y. Epub 2020 May 11. Nat Metab. 2020. PMID: 32694662
-
Vectorization of optically sectioned brain microvasculature: learning aids completion of vascular graphs by connecting gaps and deleting open-ended segments.Med Image Anal. 2012 Aug;16(6):1241-58. doi: 10.1016/j.media.2012.06.004. Epub 2012 Jun 26. Med Image Anal. 2012. PMID: 22854035 Free PMC article.
-
Neurovascular Inflammaging in Health and Disease.Cells. 2020 Jul 4;9(7):1614. doi: 10.3390/cells9071614. Cells. 2020. PMID: 32635451 Free PMC article. Review.
References
-
- Abreu-Villaça Y, Silva WC, Manhães AC, Schmidt SL. The effect of corpus callosum agenesis on neocortical thickness and neuronal density of BALB/cCF mice. Brain Res Bull. 2002;58:411–416. - PubMed
-
- Bass NH, Hess HH, Pope A, Thalheimer C. Quantitative cytoarchitectonic distribution of neurons, glia, and DNa in rat cerebral cortex. J Comp Neurol. 1971;143:481–490. - PubMed
-
- Bell MA, Ball MJ. Laminar variation in the microvascular architecture of normal human visual cortex (area 17) Brain Res. 1985;335:139–143. - PubMed
Publication types
MeSH terms
Grants and funding
- R01 DC006849/DC/NIDCD NIH HHS/United States
- NS059832/NS/NINDS NIH HHS/United States
- MH72570/MH/NIMH NIH HHS/United States
- R21 NS052565/NS/NINDS NIH HHS/United States
- R01 NS043300/NS/NINDS NIH HHS/United States
- R01 EB003832/EB/NIBIB NIH HHS/United States
- NS43300/NS/NINDS NIH HHS/United States
- MH085499/MH/NIMH NIH HHS/United States
- R01 MH085499/MH/NIMH NIH HHS/United States
- NS052565/NS/NINDS NIH HHS/United States
- DC006849/DC/NIDCD NIH HHS/United States
- R21 MH072570/MH/NIMH NIH HHS/United States
- EB003832/EB/NIBIB NIH HHS/United States
- R21 NS059832/NS/NINDS NIH HHS/United States
LinkOut - more resources
Full Text Sources
Other Literature Sources