Telomere shortening in neural stem cells disrupts neuronal differentiation and neuritogenesis
- PMID: 19923274
- PMCID: PMC6665809
- DOI: 10.1523/JNEUROSCI.3836-09.2009
Telomere shortening in neural stem cells disrupts neuronal differentiation and neuritogenesis
Abstract
Proliferation in the subependymal zone (SEZ) and neurogenesis in the olfactory bulb decline in the forebrain of telomerase-deficient mice. The present work reveals additional effects of telomere shortening on neuronal differentiation, as adult multipotent progenitors with critically short telomeres yield reduced numbers of neurons that, furthermore, exhibit underdeveloped neuritic arbors. Genetic data indicate that the tumor suppressor protein p53 not only mediates the adverse effects of telomere attrition on proliferation and self-renewal but it is also involved in preventing normal neuronal differentiation of adult progenitors with dysfunctional telomeres. Interestingly, progenitor cells with short telomeres obtained from fetal brains do not exhibit any replicative defects but also fail to acquire a fully mature neuritic arbor, demonstrating cell cycle-independent effects of telomeres on neuronal differentiation. The negative effect of p53 on neuritogenesis is mechanistically linked to its cooperation with the Notch pathway in the upregulation of small GTPase RhoA kinases, Rock1 and Rock2, suggesting a potential link between DNA damage and the Notch signaling pathway in the control of neuritogenesis. We also show that telomerase expression is downregulated in the SEZ of aging mice leading to telomere length reductions in neurosphere-forming cells and deficient neurogenesis and neuritogenesis. Our results suggest that age-related deficits could be caused partly by dysfunctional telomeres and demonstrate that p53 is a central modulator of adult neurogenesis, regulating both the production and differentiation of postnatally generated olfactory neurons.
Figures
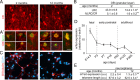
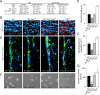
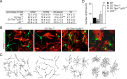
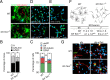
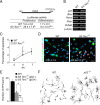
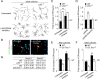
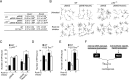
Similar articles
-
Telomerase reactivation reverses tissue degeneration in aged telomerase-deficient mice.Nature. 2011 Jan 6;469(7328):102-6. doi: 10.1038/nature09603. Epub 2010 Nov 28. Nature. 2011. PMID: 21113150 Free PMC article.
-
p53 regulates the self-renewal and differentiation of neural precursors.Neuroscience. 2009 Feb 18;158(4):1378-89. doi: 10.1016/j.neuroscience.2008.10.052. Epub 2008 Nov 7. Neuroscience. 2009. PMID: 19038313
-
Pulmonary Alveolar Stem Cell Senescence, Apoptosis, and Differentiation by p53-Dependent and -Independent Mechanisms in Telomerase-Deficient Mice.Cells. 2021 Oct 26;10(11):2892. doi: 10.3390/cells10112892. Cells. 2021. PMID: 34831112 Free PMC article.
-
Control of Cellular Aging, Tissue Function, and Cancer by p53 Downstream of Telomeres.Cold Spring Harb Perspect Med. 2017 May 1;7(5):a026088. doi: 10.1101/cshperspect.a026088. Cold Spring Harb Perspect Med. 2017. PMID: 28289249 Free PMC article. Review.
-
DNA repair factors and telomere-chromosome integrity in mammalian cells.Cytogenet Genome Res. 2004;104(1-4):116-22. doi: 10.1159/000077475. Cytogenet Genome Res. 2004. PMID: 15162024 Review.
Cited by
-
Telomeres and Telomerase in the Control of Stem Cells.Biomedicines. 2022 Sep 20;10(10):2335. doi: 10.3390/biomedicines10102335. Biomedicines. 2022. PMID: 36289597 Free PMC article. Review.
-
Acupuncture and moxibustion therapy for cognitive impairment: the microbiome-gut-brain axis and its role.Front Neurosci. 2024 Jan 11;17:1275860. doi: 10.3389/fnins.2023.1275860. eCollection 2023. Front Neurosci. 2024. PMID: 38274501 Free PMC article. Review.
-
The impact of age on oncogenic potential: tumor-initiating cells and the brain microenvironment.Aging Cell. 2013 Oct;12(5):733-41. doi: 10.1111/acel.12104. Epub 2013 Jun 28. Aging Cell. 2013. PMID: 23711239 Free PMC article. Review.
-
Targeting Cardiac Stem Cell Senescence to Treat Cardiac Aging and Disease.Cells. 2020 Jun 26;9(6):1558. doi: 10.3390/cells9061558. Cells. 2020. PMID: 32604861 Free PMC article. Review.
-
In medio stat virtus: unanticipated consequences of telomere dysequilibrium.Philos Trans R Soc Lond B Biol Sci. 2018 Mar 5;373(1741):20160444. doi: 10.1098/rstb.2016.0444. Philos Trans R Soc Lond B Biol Sci. 2018. PMID: 29335368 Free PMC article. Review.
References
-
- Alvarez-Buylla A, Lim DA. For the long run: maintaining germinal niches in the adult brain. Neuron. 2004;41:683–686. - PubMed
-
- Armesilla-Diaz A, Bragado P, Del Valle I, Cuevas E, Lazaro I, Martin C, Cigudosa JC, Silva A. p53 regulates the self-renewal and differentiation of neural precursors. Neuroscience. 2009;158:1378–1389. - PubMed
-
- Blasco MA. Telomerase beyond telomeres. Nat Rev Cancer. 2002;2:627–633. - PubMed
-
- Blasco MA, Lee HW, Hande MP, Samper E, Lansdorp PM, DePinho RA, Greider CW. Telomere shortening and tumor formation by mouse cells lacking telomerase RNA. Cell. 1997;91:25–34. - PubMed
Publication types
MeSH terms
Substances
LinkOut - more resources
Full Text Sources
Medical
Molecular Biology Databases
Research Materials
Miscellaneous