The RNA polymerase activity of SARS-coronavirus nsp12 is primer dependent
- PMID: 19875418
- PMCID: PMC2800238
- DOI: 10.1093/nar/gkp904
The RNA polymerase activity of SARS-coronavirus nsp12 is primer dependent
Erratum in
- Nucleic Acids Res. 2011 Nov;39(21):9458
Abstract
An RNA-dependent RNA polymerase (RdRp) is the central catalytic subunit of the RNA-synthesizing machinery of all positive-strand RNA viruses. Usually, RdRp domains are readily identifiable by comparative sequence analysis, but biochemical confirmation and characterization can be hampered by intrinsic protein properties and technical complications. It is presumed that replication and transcription of the approximately 30-kb severe acute respiratory syndrome (SARS) coronavirus (SARS-CoV) RNA genome are catalyzed by an RdRp domain in the C-terminal part of nonstructural protein 12 (nsp12), one of 16 replicase subunits. However, thus far full-length nsp12 has proven refractory to expression in bacterial systems, which has hindered both the biochemical characterization of coronavirus RNA synthesis and RdRp-targeted antiviral drug design. Here, we describe a combined strategy involving bacterial expression of an nsp12 fusion protein and its in vivo cleavage to generate and purify stable SARS-CoV nsp12 (106 kDa) with a natural N-terminus and C-terminal hexahistidine tag. This recombinant protein possesses robust in vitro RdRp activity, as well as a significant DNA-dependent activity that may facilitate future inhibitor studies. The SARS-CoV nsp12 is primer dependent on both homo- and heteropolymeric templates, supporting the likeliness of a close enzymatic collaboration with the intriguing RNA primase activity that was recently proposed for coronavirus nsp8.
Figures
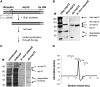
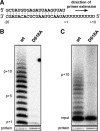
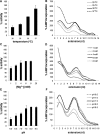
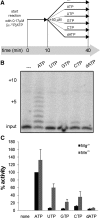
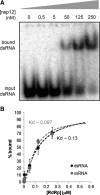
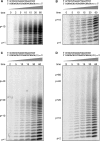
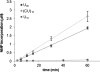
Similar articles
-
Biochemical characterization of a recombinant SARS coronavirus nsp12 RNA-dependent RNA polymerase capable of copying viral RNA templates.Arch Virol. 2012 Nov;157(11):2095-104. doi: 10.1007/s00705-012-1404-x. Epub 2012 Jul 13. Arch Virol. 2012. PMID: 22791111 Free PMC article.
-
The SARS-coronavirus nsp7+nsp8 complex is a unique multimeric RNA polymerase capable of both de novo initiation and primer extension.Nucleic Acids Res. 2012 Feb;40(4):1737-47. doi: 10.1093/nar/gkr893. Epub 2011 Oct 29. Nucleic Acids Res. 2012. PMID: 22039154 Free PMC article.
-
A second, non-canonical RNA-dependent RNA polymerase in SARS coronavirus.EMBO J. 2006 Oct 18;25(20):4933-42. doi: 10.1038/sj.emboj.7601368. Epub 2006 Oct 5. EMBO J. 2006. PMID: 17024178 Free PMC article.
-
Insights into RNA synthesis, capping, and proofreading mechanisms of SARS-coronavirus.Virus Res. 2014 Dec 19;194:90-9. doi: 10.1016/j.virusres.2014.10.008. Epub 2014 Oct 17. Virus Res. 2014. PMID: 25451065 Free PMC article. Review.
-
SARS-CoV ORF1b-encoded nonstructural proteins 12-16: replicative enzymes as antiviral targets.Antiviral Res. 2014 Jan;101:122-30. doi: 10.1016/j.antiviral.2013.11.006. Epub 2013 Nov 20. Antiviral Res. 2014. PMID: 24269475 Free PMC article. Review.
Cited by
-
Mechanism involved in the pathogenesis and immune response against SARS-CoV-2 infection.Virusdisease. 2021 Jun;32(2):211-219. doi: 10.1007/s13337-021-00687-2. Epub 2021 May 4. Virusdisease. 2021. PMID: 33969150 Free PMC article. Review.
-
Biogenesis and dynamics of the coronavirus replicative structures.Viruses. 2012 Nov 21;4(11):3245-69. doi: 10.3390/v4113245. Viruses. 2012. PMID: 23202524 Free PMC article. Review.
-
Implications of altered replication fidelity on the evolution and pathogenesis of coronaviruses.Curr Opin Virol. 2012 Oct;2(5):519-24. doi: 10.1016/j.coviro.2012.07.005. Epub 2012 Aug 1. Curr Opin Virol. 2012. PMID: 22857992 Free PMC article. Review.
-
Atlas of coronavirus replicase structure.Virus Res. 2014 Dec 19;194:49-66. doi: 10.1016/j.virusres.2013.12.004. Epub 2013 Dec 16. Virus Res. 2014. PMID: 24355834 Free PMC article. Review.
-
Nucleotide Analogues as Probes for DNA and RNA Polymerases.Curr Protoc Chem Biol. 2010;2(2):111-124. doi: 10.1002/9780470559277.ch090203. Curr Protoc Chem Biol. 2010. PMID: 21822500 Free PMC article.
References
Publication types
MeSH terms
Substances
Grants and funding
LinkOut - more resources
Full Text Sources
Other Literature Sources
Molecular Biology Databases
Research Materials
Miscellaneous