Sensing, physiological effects and molecular response to elevated CO2 levels in eukaryotes
- PMID: 19863692
- PMCID: PMC4515048
- DOI: 10.1111/j.1582-4934.2009.00952.x
Sensing, physiological effects and molecular response to elevated CO2 levels in eukaryotes
Abstract
Carbon dioxide (CO(2)) is an important gaseous molecule that maintains biosphere homeostasis and is an important cellular signalling molecule in all organisms. The transport of CO(2) through membranes has fundamental roles in most basic aspects of life in both plants and animals. There is a growing interest in understanding how CO(2) is transported into cells, how it is sensed by neurons and other cell types and in understanding the physiological and molecular consequences of elevated CO(2) levels (hypercapnia) at the cell and organism levels. Human pulmonary diseases and model organisms such as fungi, C. elegans, Drosophila and mice have been proven to be important in understanding of the mechanisms of CO(2) sensing and response.
Figures
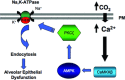
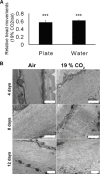
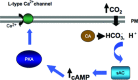
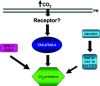
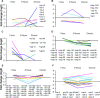
Similar articles
-
The physiological and molecular effects of elevated CO2 levels.Cell Cycle. 2010 Apr 15;9(8):1528-32. doi: 10.4161/cc.9.8.11196. Epub 2010 Apr 15. Cell Cycle. 2010. PMID: 20372066 Review.
-
Measuring the effects of high CO₂ levels in Caenorhabditis elegans.Methods. 2014 Aug 1;68(3):487-91. doi: 10.1016/j.ymeth.2014.03.008. Epub 2014 Mar 17. Methods. 2014. PMID: 24650565
-
Mechanisms and Consequences of Oxygen and Carbon Dioxide Sensing in Mammals.Physiol Rev. 2020 Jan 1;100(1):463-488. doi: 10.1152/physrev.00003.2019. Epub 2019 Sep 20. Physiol Rev. 2020. PMID: 31539306 Review.
-
Effects of hypercapnia on the lung.J Physiol. 2017 Apr 15;595(8):2431-2437. doi: 10.1113/JP273781. Epub 2017 Feb 14. J Physiol. 2017. PMID: 28044311 Free PMC article. Review.
-
CO2 sensing in fungi and beyond.Curr Opin Microbiol. 2006 Dec;9(6):572-8. doi: 10.1016/j.mib.2006.09.003. Epub 2006 Oct 11. Curr Opin Microbiol. 2006. PMID: 17045514 Review.
Cited by
-
The transcription factor Flo8 mediates CO2 sensing in the human fungal pathogen Candida albicans.Mol Biol Cell. 2012 Jul;23(14):2692-701. doi: 10.1091/mbc.E12-02-0094. Epub 2012 May 23. Mol Biol Cell. 2012. PMID: 22621896 Free PMC article.
-
Blunted behavioral and c Fos responses to acidic fumes in the African naked mole-rat.PLoS One. 2012;7(9):e45060. doi: 10.1371/journal.pone.0045060. Epub 2012 Sep 17. PLoS One. 2012. PMID: 23028761 Free PMC article.
-
Physiological carbon dioxide, bicarbonate, and pH sensing.Pflugers Arch. 2010 Nov;460(6):953-64. doi: 10.1007/s00424-010-0865-6. Epub 2010 Aug 4. Pflugers Arch. 2010. PMID: 20683624 Free PMC article. Review.
-
Imaginal disk growth factors are Drosophila chitinase-like proteins with roles in morphogenesis and CO2 response.Genetics. 2023 Feb 9;223(2):iyac185. doi: 10.1093/genetics/iyac185. Genetics. 2023. PMID: 36576887 Free PMC article.
-
Comparative Transcriptomics of Flammulina filiformis Suggests a High CO2 Concentration Inhibits Early Pileus Expansion by Decreasing Cell Division Control Pathways.Int J Mol Sci. 2019 Nov 25;20(23):5923. doi: 10.3390/ijms20235923. Int J Mol Sci. 2019. PMID: 31775357 Free PMC article.
References
Publication types
MeSH terms
Substances
Grants and funding
LinkOut - more resources
Full Text Sources
Molecular Biology Databases