Structural relationships among proteins with different global topologies and their implications for function annotation strategies
- PMID: 19805138
- PMCID: PMC2765090
- DOI: 10.1073/pnas.0907971106
Structural relationships among proteins with different global topologies and their implications for function annotation strategies
Abstract
It has become increasingly apparent that geometric relationships often exist between regions of two proteins that have quite different global topologies or folds. In this article, we examine whether such relationships can be used to infer a functional connection between the two proteins in question. We find, by considering a number of examples involving metal and cation binding, sugar binding, and aromatic group binding, that geometrically similar protein fragments can share related functions, even if they have been classified as belonging to different folds and topologies. Thus, the use of classifications inevitably limits the number of functional inferences that can be obtained from the comparative analysis of protein structures. In contrast, the development of interactive computational tools that recognize the "continuous" nature of protein structure/function space, by increasing the number of potentially meaningful relationships that are considered, may offer a dramatic enhancement in the ability to extract information from protein structure databases. We introduce the MarkUs server, that embodies this strategy and that is designed for a user interested in developing and validating specific functional hypotheses.
Conflict of interest statement
The authors declare no conflict of interest.
Figures
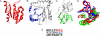
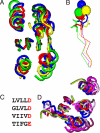
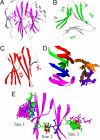
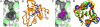
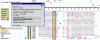
Similar articles
-
Template-Based Prediction of Protein-Peptide Interactions by Using GalaxyPepDock.Methods Mol Biol. 2017;1561:37-47. doi: 10.1007/978-1-4939-6798-8_4. Methods Mol Biol. 2017. PMID: 28236232
-
Protein functional annotation by homology.Methods Mol Biol. 2008;484:465-90. doi: 10.1007/978-1-59745-398-1_28. Methods Mol Biol. 2008. PMID: 18592196
-
Structural motifs recurring in different folds recognize the same ligand fragments.BMC Bioinformatics. 2009 Jun 15;10:182. doi: 10.1186/1471-2105-10-182. BMC Bioinformatics. 2009. PMID: 19527512 Free PMC article.
-
NMR for the design of functional mimetics of protein-protein interactions: one key is in the building of bridges.Biochem Cell Biol. 1998;76(2-3):177-88. doi: 10.1139/bcb-76-2-3-177. Biochem Cell Biol. 1998. PMID: 9923687 Review.
-
Contemporary approaches to protein structure classification.Bioessays. 1998 Nov;20(11):884-91. doi: 10.1002/(SICI)1521-1878(199811)20:11<884::AID-BIES3>3.0.CO;2-H. Bioessays. 1998. PMID: 9872054 Review.
Cited by
-
CLIPS-1D: analysis of multiple sequence alignments to deduce for residue-positions a role in catalysis, ligand-binding, or protein structure.BMC Bioinformatics. 2012 Apr 5;13:55. doi: 10.1186/1471-2105-13-55. BMC Bioinformatics. 2012. PMID: 22480135 Free PMC article.
-
The SHOCT domain: a widespread domain under-represented in model organisms.PLoS One. 2013;8(2):e57848. doi: 10.1371/journal.pone.0057848. Epub 2013 Feb 25. PLoS One. 2013. PMID: 23451277 Free PMC article.
-
Solution NMR structure of photosystem II reaction center protein Psb28 from Synechocystis sp. Strain PCC 6803.Proteins. 2011 Jan;79(1):340-4. doi: 10.1002/prot.22876. Proteins. 2011. PMID: 21058299 Free PMC article. No abstract available.
-
Interplay of physics and evolution in the likely origin of protein biochemical function.Proc Natl Acad Sci U S A. 2013 Jun 4;110(23):9344-9. doi: 10.1073/pnas.1300011110. Epub 2013 May 20. Proc Natl Acad Sci U S A. 2013. PMID: 23690621 Free PMC article.
-
Solution NMR structure of VF0530 from Vibrio fischeri reveals a nucleic acid-binding function.Proteins. 2011 Oct;79(10):2988-91. doi: 10.1002/prot.23121. Epub 2011 Aug 26. Proteins. 2011. PMID: 21905121 Free PMC article.
References
-
- Yang AS, Honig B. An integrated approach to the analysis and modeling of protein sequences and structures. I. Protein structural alignment and a quantitative measure for protein structural distance. J Mol Biol. 2000;301:665–678. - PubMed
-
- Shindyalov IN, Bourne PE. An alternative view of protein fold space. Prot: Struct Func Gen. 2000;38:247–260. - PubMed
-
- Kihara D, Skolnick J. The PDB is a covering set of small protein structures. J Mol Biol. 2003;334:793–802. - PubMed
-
- Szustakowski JD, Kasif S, Weng Z. Less is more: Towards an optimal universal description of protein folds. Bioinformatics. 2005;21:ii66–71. - PubMed
-
- Friedberg I, Godzik A. Connecting the protein structure universe by using sparse recurring fragments. Structure. 2005;13:1213–1224. - PubMed
Publication types
MeSH terms
Substances
Grants and funding
LinkOut - more resources
Full Text Sources
Other Literature Sources