Sustained oscillations of NF-kappaB produce distinct genome scanning and gene expression profiles
- PMID: 19787057
- PMCID: PMC2747007
- DOI: 10.1371/journal.pone.0007163
Sustained oscillations of NF-kappaB produce distinct genome scanning and gene expression profiles
Abstract
NF-kappaB is a prototypic stress-responsive transcription factor that acts within a complex regulatory network. The signaling dynamics of endogenous NF-kappaB in single cells remain poorly understood. To examine real time dynamics in living cells, we monitored NF-kappaB activities at multiple timescales using GFP-p65 knock-in mouse embryonic fibroblasts. Oscillations in NF-kappaB were sustained in most cells, with several cycles of transient nuclear translocation after TNF-alpha stimulation. Mathematical modeling suggests that NF-kappaB oscillations are selected over other non-oscillatory dynamics by fine-tuning the relative strengths of feedback loops like IkappaBalpha. The ability of NF-kappaB to scan and interact with the genome in vivo remained remarkably constant from early to late cycles, as observed by fluorescence recovery after photobleaching (FRAP). Perturbation of long-term NF-kappaB oscillations interfered with its short-term interaction with chromatin and balanced transcriptional output, as predicted by the mathematical model. We propose that negative feedback loops do not simply terminate signaling, but rather promote oscillations of NF-kappaB in the nucleus, and these oscillations are functionally advantageous.
Conflict of interest statement
Figures
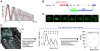
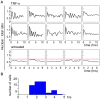
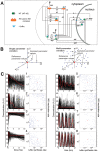
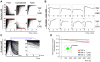
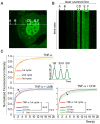
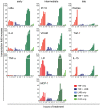
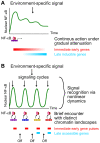
Similar articles
-
High-throughput analysis of NF-κB dynamics in single cells reveals basal nuclear localization of NF-κB and spontaneous activation of oscillations.PLoS One. 2014 Mar 4;9(3):e90104. doi: 10.1371/journal.pone.0090104. eCollection 2014. PLoS One. 2014. PMID: 24595030 Free PMC article.
-
IkappaBepsilon provides negative feedback to control NF-kappaB oscillations, signaling dynamics, and inflammatory gene expression.J Cell Biol. 2006 Jun 5;173(5):659-64. doi: 10.1083/jcb.200510155. Epub 2006 May 30. J Cell Biol. 2006. PMID: 16735576 Free PMC article.
-
Oscillations in NF-kappaB signaling control the dynamics of gene expression.Science. 2004 Oct 22;306(5696):704-8. doi: 10.1126/science.1099962. Science. 2004. PMID: 15499023
-
A TNF-induced gene expression program under oscillatory NF-kappaB control.BMC Genomics. 2005 Sep 28;6:137. doi: 10.1186/1471-2164-6-137. BMC Genomics. 2005. PMID: 16191192 Free PMC article.
-
Regulation of Transcription Factor NF-κB in Its Natural Habitat: The Nucleus.Cells. 2021 Mar 29;10(4):753. doi: 10.3390/cells10040753. Cells. 2021. PMID: 33805563 Free PMC article. Review.
Cited by
-
Exploring the role of macromolecular crowding and TNFR1 in cell volume control.Elife. 2024 Sep 19;13:e92719. doi: 10.7554/eLife.92719. Elife. 2024. PMID: 39297502 Free PMC article.
-
Cell shape and the microenvironment regulate nuclear translocation of NF-κB in breast epithelial and tumor cells.Mol Syst Biol. 2015 Mar;11(3):790. doi: 10.15252/msb.20145644. Mol Syst Biol. 2015. PMID: 26148352 Free PMC article.
-
Roles of spatial parameters on the oscillation of nuclear NF-κB: computer simulations of a 3D spherical cell.PLoS One. 2012;7(10):e46911. doi: 10.1371/journal.pone.0046911. Epub 2012 Oct 3. PLoS One. 2012. PMID: 23056526 Free PMC article.
-
A method of ‘speed coefficients’ for biochemical model reduction applied to the NF-κB system.J Math Biol. 2015 Feb;70(3):591-620. doi: 10.1007/s00285-014-0775-x. J Math Biol. 2015. PMID: 24658784 Free PMC article.
-
Requirements for mammalian promoters to decode transcription factor dynamics.Nucleic Acids Res. 2023 May 22;51(9):4674-4690. doi: 10.1093/nar/gkad273. Nucleic Acids Res. 2023. PMID: 37070176 Free PMC article.
References
-
- Hoffmann A, Levchenko A, Scott ML, Baltimore D. The IkappaB-NF-kappaB signaling module: temporal control and selective gene activation. Science. 2002;298:1241–1245. - PubMed
-
- Nelson DE, Ihekwaba AE, Elliott M, Johnson JR, Gibney CA, et al. Oscillations in NF-kappaB signaling control the dynamics of gene expression. Science. 2004;306:704–708. - PubMed
-
- Hayden MS, Ghosh S. Shared principles in NF-kappaB signaling. Cell. 2008;132:344–362. - PubMed
-
- Cheong R, Bergmann A, Werner SL, Regal J, Hoffmann A, et al. Transient IkappaB kinase activity mediates temporal NF-kappaB dynamics in response to a wide range of tumor necrosis factor-alpha doses. J Biol Chem. 2006;281:2945–2950. - PubMed
Publication types
MeSH terms
Substances
Grants and funding
LinkOut - more resources
Full Text Sources
Other Literature Sources
Molecular Biology Databases
Miscellaneous