Structural basis of HIV-1 activation by NF-kappaB--a higher-order complex of p50:RelA bound to the HIV-1 LTR
- PMID: 19683540
- PMCID: PMC2753696
- DOI: 10.1016/j.jmb.2009.08.023
Structural basis of HIV-1 activation by NF-kappaB--a higher-order complex of p50:RelA bound to the HIV-1 LTR
Abstract
The activation and latency of human immunodeficiency virus type 1 (HIV-1) are tightly controlled by the transcriptional activity of its long terminal repeat (LTR) region. The LTR is regulated by viral proteins as well as host factors, including the nuclear factor kappaB (NF-kappaB) that becomes activated in virus-infected cells. The two tandem NF-kappaB sites of the LTR are among the most highly conserved sequence elements of the HIV-1 genome. Puzzlingly, these sites are arranged in a manner that seems to preclude simultaneous binding of both sites by NF-kappaB, although previous biochemical work suggests otherwise. Here, we have determined the crystal structure of p50:RelA bound to the tandem kappaB element of the HIV-1 LTR as a dimeric dimer, providing direct structural evidence that NF-kappaB can occupy both sites simultaneously. The two p50:RelA dimers bind the adjacent kappaB sites and interact through a protein contact that is accommodated by DNA bending. The two dimers clamp DNA from opposite faces of the double helix and form a topological trap of the bound DNA. Consistent with these structural features, our biochemical analyses indicate that p50:RelA binds the HIV-1 LTR tandem kappaB sites with an apparent anti-cooperativity but enhanced kinetic stability. The slow on and off rates we observe may be relevant to viral latency because viral activation requires sustained NF-kappaB activation. Furthermore, our work demonstrates that the specific arrangement of the two kappaB sites on the HIV-1 LTR can modulate the assembly kinetics of the higher-order NF-kappaB complex on the viral promoter. This phenomenon is unlikely restricted to the HIV-1 LTR but probably represents a general mechanism for the function of composite DNA elements in transcription.
Figures
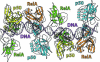
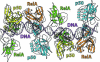
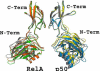
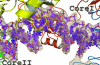
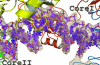
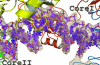
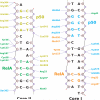
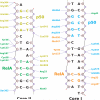
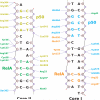
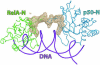
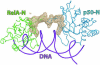
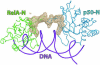
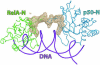
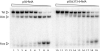
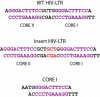
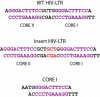
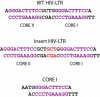
Similar articles
-
Double plant homeodomain (PHD) finger proteins DPF3a and -3b are required as transcriptional co-activators in SWI/SNF complex-dependent activation of NF-κB RelA/p50 heterodimer.J Biol Chem. 2012 Apr 6;287(15):11924-33. doi: 10.1074/jbc.M111.322792. Epub 2012 Feb 13. J Biol Chem. 2012. PMID: 22334708 Free PMC article.
-
Negative regulation of HIV-1 transcription by a heterodimeric NF-κB1/p50 and C-terminally truncated STAT5 complex.J Mol Biol. 2011 Jul 29;410(5):933-43. doi: 10.1016/j.jmb.2011.03.044. J Mol Biol. 2011. PMID: 21763497
-
Regulation of HIV-1 long terminal repeats by interaction of C/EBP(NF-IL6) and NF-kappaB/Rel transcription factors.J Biol Chem. 1996 Sep 13;271(37):22479-86. doi: 10.1074/jbc.271.37.22479. J Biol Chem. 1996. PMID: 8798413
-
The multifunctional role of intrinsic disorder in NF-κB signaling.Biochem Soc Trans. 2023 Dec 20;51(6):2085-2092. doi: 10.1042/BST20230035. Biochem Soc Trans. 2023. PMID: 38095058 Free PMC article. Review.
-
DNA topoisomerase 1 represses HIV-1 promoter activity through its interaction with a guanine quadruplex present in the LTR sequence.Retrovirology. 2023 May 30;20(1):10. doi: 10.1186/s12977-023-00625-8. Retrovirology. 2023. PMID: 37254203 Free PMC article. Review.
Cited by
-
Exploring Potentilla nepalensis Phytoconstituents: Integrated Strategies of Network Pharmacology, Molecular Docking, Dynamic Simulations, and MMGBSA Analysis for Cancer Therapeutic Targets Discovery.Pharmaceuticals (Basel). 2024 Jan 19;17(1):134. doi: 10.3390/ph17010134. Pharmaceuticals (Basel). 2024. PMID: 38276007 Free PMC article.
-
Computational evaluation of bioactive compounds in Curcuma zanthorrhiza targeting SIRT1 and NFκB.BioTechnologia (Pozn). 2023 Jun 26;104(2):171-182. doi: 10.5114/bta.2023.127206. eCollection 2023. BioTechnologia (Pozn). 2023. PMID: 37427032 Free PMC article.
-
Epigenetics, N-myrystoyltransferase-1 and casein kinase-2-alpha modulates the increased replication of HIV-1 CRF02_AG, compared to subtype-B viruses.Sci Rep. 2019 Jul 23;9(1):10689. doi: 10.1038/s41598-019-47069-9. Sci Rep. 2019. PMID: 31337802 Free PMC article.
-
HTLV-1, the Other Pathogenic Yet Neglected Human Retrovirus: From Transmission to Therapeutic Treatment.Viruses. 2017 Dec 21;10(1):1. doi: 10.3390/v10010001. Viruses. 2017. PMID: 29267225 Free PMC article. Review.
-
xCT/SLC7A11 antiporter function inhibits HIV-1 infection.Virology. 2021 Apr;556:149-160. doi: 10.1016/j.virol.2021.01.008. Epub 2021 Jan 20. Virology. 2021. PMID: 33631414 Free PMC article.
References
-
- Nabel G, Baltimore D. An inducible transcription factor activates expression of human immunodeficiency virus in T cells. Nature. 1987;326:711–3. - PubMed
-
- Siekevitz M, Josephs SF, Dukovich M, Peffer N, Wong-Staal F, Greene WC. Activation of the HIV-1 LTR by T cell mitogens and the trans-activator protein of HTLV-I. Science. 1987;238:1575–8. - PubMed
-
- Levine BL, Mosca JD, Riley JL, Carroll RG, Vahey MT, Jagodzinski LL, Wagner KF, Mayers DL, Burke DS, Weislow OS, Louis DC, June CH. Antiviral effect and ex vivo CD4+ T cell proliferation in HIV-positive patients as a result of CD28 costimulation. Science. 1996;272:1939–43. - PubMed
Publication types
MeSH terms
Substances
Associated data
- Actions
Grants and funding
LinkOut - more resources
Full Text Sources
Molecular Biology Databases
Research Materials