Collagen-based cell migration models in vitro and in vivo
- PMID: 19682592
- PMCID: PMC4021709
- DOI: 10.1016/j.semcdb.2009.08.005
Collagen-based cell migration models in vitro and in vivo
Abstract
Fibrillar collagen is the most abundant extracellular matrix (ECM) constituent which maintains the structure of most interstitial tissues and organs, including skin, gut, and breast. Density and spatial alignments of the three-dimensional (3D) collagen architecture define mechanical tissue properties, i.e. stiffness and porosity, which guide or oppose cell migration and positioning in different contexts, such as morphogenesis, regeneration, immune response, and cancer progression. To reproduce interstitial cell movement in vitro with high in vivo fidelity, 3D collagen lattices are being reconstituted from extracted collagen monomers, resulting in the re-assembly of a fibrillar meshwork of defined porosity and stiffness. With a focus on tumor invasion studies, we here evaluate different in vitro collagen-based cell invasion models, employing either pepsinized or non-pepsinized collagen extracts, and compare their structure to connective tissue in vivo, including mouse dermis and mammary gland, chick chorioallantoic membrane (CAM), and human dermis. Using confocal reflection and two-photon-excited second harmonic generation (SHG) microscopy, we here show that, depending on the collagen source, in vitro models yield homogeneous fibrillar texture with a quite narrow range of pore size variation, whereas all in vivo scaffolds comprise a range from low- to high-density fibrillar networks and heterogeneous pore sizes within the same tissue. Future in-depth comparison of structure and physical properties between 3D ECM-based models in vitro and in vivo are mandatory to better understand the mechanisms and limits of interstitial cell movements in distinct tissue environments.
Figures
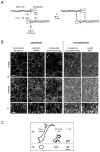
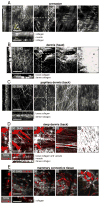
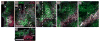
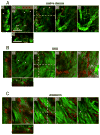
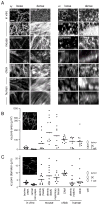
Similar articles
-
Time-lapse confocal reflection microscopy of collagen fibrillogenesis and extracellular matrix assembly in vitro.Biopolymers. 2000 Sep;54(3):222-34. doi: 10.1002/1097-0282(200009)54:3<222::AID-BIP80>3.0.CO;2-K. Biopolymers. 2000. PMID: 10861383
-
Mammary fibroblasts remodel fibrillar collagen microstructure in a biomimetic nanocomposite hydrogel.Acta Biomater. 2019 Jan 1;83:221-232. doi: 10.1016/j.actbio.2018.11.010. Epub 2018 Nov 7. Acta Biomater. 2019. PMID: 30414485 Free PMC article.
-
Physical network regimes of 3D fibrillar collagen networks trigger invasive phenotypes of breast cancer cells.Biomater Adv. 2024 Oct;163:213961. doi: 10.1016/j.bioadv.2024.213961. Epub 2024 Jul 16. Biomater Adv. 2024. PMID: 39032434
-
Biomimetic tumor microenvironments based on collagen matrices.Biomater Sci. 2018 Jul 24;6(8):2009-2024. doi: 10.1039/c8bm00303c. Biomater Sci. 2018. PMID: 29999062 Review.
-
Multiscale Label-Free Imaging of Fibrillar Collagen in the Tumor Microenvironment.Methods Mol Biol. 2023;2614:187-235. doi: 10.1007/978-1-0716-2914-7_13. Methods Mol Biol. 2023. PMID: 36587127 Review.
Cited by
-
Cell migration.Compr Physiol. 2012 Oct;2(4):2369-92. doi: 10.1002/cphy.c110012. Compr Physiol. 2012. PMID: 23720251 Free PMC article. Review.
-
Tunable collagen I hydrogels for engineered physiological tissue micro-environments.PLoS One. 2015 Mar 30;10(3):e0122500. doi: 10.1371/journal.pone.0122500. eCollection 2015. PLoS One. 2015. PMID: 25822731 Free PMC article.
-
Mechanical stress during confined migration causes aberrant mitoses and c-MYC amplification.Proc Natl Acad Sci U S A. 2024 Jul 16;121(29):e2404551121. doi: 10.1073/pnas.2404551121. Epub 2024 Jul 11. Proc Natl Acad Sci U S A. 2024. PMID: 38990945 Free PMC article.
-
Cytoplasmic accumulation and plasma membrane association of anillin and Ect2 promote confined migration and invasion.Res Sq [Preprint]. 2024 Jan 10:rs.3.rs-3640969. doi: 10.21203/rs.3.rs-3640969/v1. Res Sq. 2024. PMID: 38260442 Free PMC article. Preprint.
-
The dermatan sulfate proteoglycan decorin modulates α2β1 integrin and the vimentin intermediate filament system during collagen synthesis.PLoS One. 2012;7(12):e50809. doi: 10.1371/journal.pone.0050809. Epub 2012 Dec 3. PLoS One. 2012. PMID: 23226541 Free PMC article.
References
-
- Elbjeirami WM, Yonter EO, Starcher BC, West JL. Enhancing mechanical properties of tissue-engineered constructs via lysyl oxidase crosslinking activity. J Biomed Mater Res A. 2003;66:513–21. - PubMed
-
- Paszek MJ, Zahir N, Johnson KR, Lakins JN, Rozenberg GI, Gefen A, et al. Tensional homeostasis and the malignant phenotype. Cancer Cell. 2005;8:241–54. - PubMed
-
- Daley WP, Peters SB, Larsen M. Extracellular matrix dynamics in development and regenerative medicine. J Cell Sci. 2008;121:255–64. - PubMed
Publication types
MeSH terms
Substances
Grants and funding
LinkOut - more resources
Full Text Sources
Other Literature Sources