FG/FxFG as well as GLFG repeats form a selective permeability barrier with self-healing properties
- PMID: 19680227
- PMCID: PMC2728434
- DOI: 10.1038/emboj.2009.199
FG/FxFG as well as GLFG repeats form a selective permeability barrier with self-healing properties
Abstract
The permeability barrier of nuclear pore complexes (NPCs) controls all nucleo-cytoplasmic exchange. It is freely permeable for small molecules. Objects larger than approximately 30 kDa can efficiently cross this barrier only when bound to nuclear transport receptors (NTRs) that confer translocation-promoting properties. We had shown earlier that the permeability barrier can be reconstituted in the form of a saturated FG/FxFG repeat hydrogel. We now show that GLFG repeats, the other major FG repeat type, can also form highly selective hydrogels. While supporting massive, reversible importin-mediated cargo influx, FG/FxFG, GLFG or mixed hydrogels remained firm barriers towards inert objects that lacked nuclear transport signals. This indicates that FG hydrogels immediately reseal behind a translocating species and thus possess 'self-healing' properties. NTRs not only left the barrier intact, they even tightened it against passive influx, pointing to a role for NTRs in establishing and maintaining the permeability barrier of NPCs.
Conflict of interest statement
The authors declare that they have no conflict of interest.
Figures
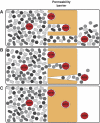
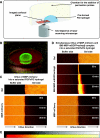
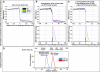
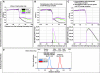
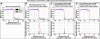
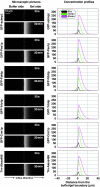
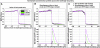
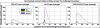
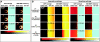
Similar articles
-
Systematic analysis of barrier-forming FG hydrogels from Xenopus nuclear pore complexes.EMBO J. 2013 Jan 23;32(2):204-18. doi: 10.1038/emboj.2012.302. Epub 2012 Nov 30. EMBO J. 2013. PMID: 23202855 Free PMC article.
-
A saturated FG-repeat hydrogel can reproduce the permeability properties of nuclear pore complexes.Cell. 2007 Aug 10;130(3):512-23. doi: 10.1016/j.cell.2007.06.024. Cell. 2007. PMID: 17693259
-
Characterisation of the passive permeability barrier of nuclear pore complexes.EMBO J. 2009 Sep 2;28(17):2541-53. doi: 10.1038/emboj.2009.200. Epub 2009 Aug 13. EMBO J. 2009. PMID: 19680228 Free PMC article.
-
The selective permeability barrier in the nuclear pore complex.Nucleus. 2016 Sep 2;7(5):430-446. doi: 10.1080/19491034.2016.1238997. Epub 2016 Sep 27. Nucleus. 2016. PMID: 27673359 Free PMC article. Review.
-
Biomechanics of the transport barrier in the nuclear pore complex.Semin Cell Dev Biol. 2017 Aug;68:42-51. doi: 10.1016/j.semcdb.2017.05.007. Epub 2017 May 12. Semin Cell Dev Biol. 2017. PMID: 28506890 Review.
Cited by
-
Conserved spatial organization of FG domains in the nuclear pore complex.Biophys J. 2013 Jan 8;104(1):37-50. doi: 10.1016/j.bpj.2012.11.3823. Epub 2013 Jan 8. Biophys J. 2013. PMID: 23332057 Free PMC article.
-
Molecular interaction mechanisms of glycol chitosan self-healing hydrogel as a drug delivery system for gemcitabine and doxorubicin.Comput Struct Biotechnol J. 2022 Jan 25;20:700-709. doi: 10.1016/j.csbj.2022.01.013. eCollection 2022. Comput Struct Biotechnol J. 2022. PMID: 35140889 Free PMC article.
-
Single-molecule studies of nucleocytoplasmic transport: from one dimension to three dimensions.Integr Biol (Camb). 2012 Jan;4(1):10-21. doi: 10.1039/c1ib00041a. Epub 2011 Oct 24. Integr Biol (Camb). 2012. PMID: 22020388 Free PMC article. Review.
-
Permeating the nuclear pore complex.Nucleus. 2010 Nov-Dec;1(6):475-80. doi: 10.4161/nucl.1.6.13112. Epub 2010 Jul 22. Nucleus. 2010. PMID: 21327089 Free PMC article.
-
A physical model describing the interaction of nuclear transport receptors with FG nucleoporin domain assemblies.Elife. 2016 Apr 8;5:e14119. doi: 10.7554/eLife.14119. Elife. 2016. PMID: 27058170 Free PMC article.
References
-
- Akey CW (1992) The nuclear pore complex: a macromolecular transport machine. In Nuclear Trafficking, Feldherr, CM (ed), pp 31–70. New York: Academic Press
-
- Bayliss R, Littlewood T, Stewart M (2000) Structural basis for the interaction between FxFG nucleoporin repeats and importin-beta in nuclear trafficking. Cell 102: 99–108 - PubMed
-
- Bayliss R, Ribbeck K, Akin D, Kent HM, Feldherr CM, Görlich D, Stewart M (1999) Interaction between NTF2 and xFxFG-containing nucleoporins is required to mediate nuclear import of RanGDP. J Mol Biol 293: 579–593 - PubMed
Publication types
MeSH terms
Substances
LinkOut - more resources
Full Text Sources
Other Literature Sources
Molecular Biology Databases