Molecular dissection of the prototype foamy virus (PFV) RNA 5'-UTR identifies essential elements of a ribosomal shunt
- PMID: 19638424
- PMCID: PMC2761275
- DOI: 10.1093/nar/gkp609
Molecular dissection of the prototype foamy virus (PFV) RNA 5'-UTR identifies essential elements of a ribosomal shunt
Abstract
The prototype foamy virus (PFV) is a nonpathogenic retrovirus that shows promise as a vector for gene transfer. The PFV (pre)genomic RNA starts with a long complex leader that can be folded into an elongated hairpin, suggesting an alternative strategy to cap-dependent linear scanning for translation initiation of the downstream GAG open reading frame (ORF). We found that the PFV leader carries several short ORFs (sORFs), with the three 5'-proximal sORFs located upstream of a structural element. Scanning-inhibitory hairpin insertion analysis suggested a ribosomal shunt mechanism, whereby ribosomes start scanning at the leader 5'-end and initiate at the downstream ORF via bypass of the central leader regions, which are inhibitory for scanning. We show that the efficiency of shunting depends strongly on the stability of the structural element located downstream of either sORFs A/A' or sORF B, and on the translation event at the corresponding 5'-proximal sORF. The PFV shunting strategy mirrors that of Cauliflower mosaic virus in plants; however, in mammals shunting can operate in the presence of a less stable structural element, although it is greatly improved by increasing the number of base pairings. At least one shunt configuration was found in primate FV (pre)genomic RNAs.
Figures
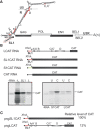
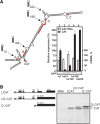
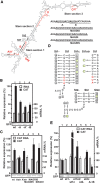
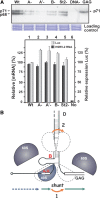
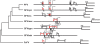
Similar articles
-
Role of a short open reading frame in ribosome shunt on the cauliflower mosaic virus RNA leader.J Biol Chem. 2000 Jun 9;275(23):17288-96. doi: 10.1074/jbc.M001143200. J Biol Chem. 2000. PMID: 10747993
-
Continuous and discontinuous ribosome scanning on the cauliflower mosaic virus 35 S RNA leader is controlled by short open reading frames.J Biol Chem. 2000 Nov 24;275(47):37278-84. doi: 10.1074/jbc.M004909200. J Biol Chem. 2000. PMID: 10973961
-
Ribosome shunting in the cauliflower mosaic virus 35S RNA leader is a special case of reinitiation of translation functioning in plant and animal systems.Genes Dev. 2000 Apr 1;14(7):817-29. Genes Dev. 2000. PMID: 10766738 Free PMC article.
-
Translational control by 5'-untranslated regions of eukaryotic mRNAs.Science. 2016 Jun 17;352(6292):1413-6. doi: 10.1126/science.aad9868. Science. 2016. PMID: 27313038 Free PMC article. Review.
-
Functional analysis of structural motifs in dicistroviruses.Virus Res. 2009 Feb;139(2):137-47. doi: 10.1016/j.virusres.2008.06.006. Epub 2008 Jul 25. Virus Res. 2009. PMID: 18621089 Review.
Cited by
-
Reselection of a genomic upstream open reading frame in mouse hepatitis coronavirus 5'-untranslated-region mutants.J Virol. 2014 Jan;88(2):846-58. doi: 10.1128/JVI.02831-13. Epub 2013 Oct 30. J Virol. 2014. PMID: 24173235 Free PMC article.
-
Host-like RNA Elements Regulate Virus Translation.Viruses. 2024 Mar 20;16(3):468. doi: 10.3390/v16030468. Viruses. 2024. PMID: 38543832 Free PMC article. Review.
-
Foamy Virus Protein-Nucleic Acid Interactions during Particle Morphogenesis.Viruses. 2016 Aug 30;8(9):243. doi: 10.3390/v8090243. Viruses. 2016. PMID: 27589786 Free PMC article. Review.
-
Revisiting the Non-Coding Nature of Pospiviroids.Cells. 2022 Jan 13;11(2):265. doi: 10.3390/cells11020265. Cells. 2022. PMID: 35053381 Free PMC article.
-
Ribosomal control in RNA virus-infected cells.Front Microbiol. 2022 Nov 7;13:1026887. doi: 10.3389/fmicb.2022.1026887. eCollection 2022. Front Microbiol. 2022. PMID: 36419416 Free PMC article. Review.
References
-
- Kozak M. Initiation of translation in prokaryotes and eukaryotes. Gene. 1999;234:187–208. - PubMed
-
- Pestova T, Lorsch JR, Hellen CUT. The mechanism of translation initiation in eukaryotes. In: Mathews MB, Sonenberg N, Hershey JWB, editors. Translational Control in Biology and Medicine. NY: Cold Spring Harbor; 2007. pp. 87–128.
-
- Jackson R. Alternative mechanisms of initiating translation of mammalian mRNAs. Biochem. Soc. Trans. 2005;33:1231–1241. - PubMed
-
- Ryabova LA, Pooggin MM, Hohn T. Translation reinitiation and leaky scanning in plant viruses. Virus Res. 2006;119:52–62. - PubMed
Publication types
MeSH terms
Substances
LinkOut - more resources
Full Text Sources
Miscellaneous