Axonal sodium channel distribution shapes the depolarized action potential threshold of dentate granule neurons
- PMID: 19603521
- PMCID: PMC2975957
- DOI: 10.1002/hipo.20667
Axonal sodium channel distribution shapes the depolarized action potential threshold of dentate granule neurons
Abstract
Intrinsic excitability is a key feature dictating neuronal response to synaptic input. Here we investigate the recent observation that dentate granule neurons exhibit a more depolarized voltage threshold for action potential initiation than CA3 pyramidal neurons. We find no evidence that tonic GABA currents, leak or voltage-gated potassium conductances, or the expression of sodium channel isoform differences can explain this depolarized threshold. Axonal initial segment voltage-gated sodium channels, which are dominated by the Na(V)1.6 isoform in both cell types, distribute more proximally and exhibit lower overall density in granule neurons than in CA3 neurons. To test possible contributions of sodium channel distributions to voltage threshold and to test whether morphological differences participate, we performed simulations of dentate granule neurons and of CA3 pyramidal neurons. These simulations revealed that cell morphology and sodium channel distribution combine to yield the characteristic granule neuron action potential upswing and voltage threshold. Proximal axon sodium channel distribution strongly contributes to the higher voltage threshold of dentate granule neurons for two reasons. First, action potential initiation closer to the somatodendritic current sink causes the threshold of the initiating axon compartment to rise. Second, the proximity of the action potential initiation site to the recording site causes somatic recordings to more faithfully reflect the depolarized threshold of the axon than in cells like CA3 neurons, with distally initiating action potentials. Our results suggest that the proximal location of axon sodium channels in dentate granule neurons contributes to the intrinsic excitability differences between DG and CA3 neurons and may participate in the low-pass filtering function of dentate granule neurons.
(c) 2009 Wiley-Liss, Inc.
Figures
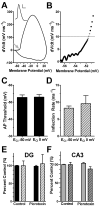
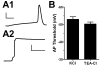
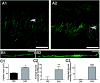
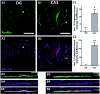
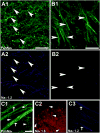
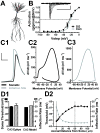
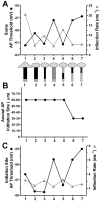
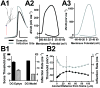
Similar articles
-
Differential effects of axon initial segment and somatodendritic GABAA receptors on excitability measures in rat dentate granule neurons.J Neurophysiol. 2011 Jan;105(1):366-79. doi: 10.1152/jn.00165.2010. Epub 2010 Nov 10. J Neurophysiol. 2011. PMID: 21068264 Free PMC article.
-
High threshold, proximal initiation, and slow conduction velocity of action potentials in dentate granule neuron mossy fibers.J Neurophysiol. 2008 Jul;100(1):281-91. doi: 10.1152/jn.90295.2008. Epub 2008 May 14. J Neurophysiol. 2008. PMID: 18480368 Free PMC article.
-
Autonomous initiation and propagation of action potentials in neurons of the subthalamic nucleus.J Physiol. 2008 Dec 1;586(23):5679-700. doi: 10.1113/jphysiol.2008.155861. Epub 2008 Oct 2. J Physiol. 2008. PMID: 18832425 Free PMC article.
-
The CA3 "backprojection" to the dentate gyrus.Prog Brain Res. 2007;163:627-37. doi: 10.1016/S0079-6123(07)63034-9. Prog Brain Res. 2007. PMID: 17765742 Free PMC article. Review.
-
Neuronal signaling in central nervous system.Sheng Li Xue Bao. 2011 Feb 25;63(1):1-8. Sheng Li Xue Bao. 2011. PMID: 21340428 Review.
Cited by
-
Fast sodium channel gating supports localized and efficient axonal action potential initiation.J Neurosci. 2010 Jul 28;30(30):10233-42. doi: 10.1523/JNEUROSCI.6335-09.2010. J Neurosci. 2010. PMID: 20668206 Free PMC article.
-
Building and maintaining the axon initial segment.Curr Opin Neurobiol. 2010 Aug;20(4):481-8. doi: 10.1016/j.conb.2010.04.012. Epub 2010 May 27. Curr Opin Neurobiol. 2010. PMID: 20537529 Free PMC article. Review.
-
Differential effects of axon initial segment and somatodendritic GABAA receptors on excitability measures in rat dentate granule neurons.J Neurophysiol. 2011 Jan;105(1):366-79. doi: 10.1152/jn.00165.2010. Epub 2010 Nov 10. J Neurophysiol. 2011. PMID: 21068264 Free PMC article.
-
Structural and Functional Plasticity at the Axon Initial Segment.Front Cell Neurosci. 2016 Oct 25;10:250. doi: 10.3389/fncel.2016.00250. eCollection 2016. Front Cell Neurosci. 2016. PMID: 27826229 Free PMC article. Review.
-
Diverse impact of acute and long-term extracellular proteolytic activity on plasticity of neuronal excitability.Front Cell Neurosci. 2015 Aug 10;9:313. doi: 10.3389/fncel.2015.00313. eCollection 2015. Front Cell Neurosci. 2015. PMID: 26321914 Free PMC article. Review.
References
-
- Bean BP. The action potential in mammalian central neurons. Nat Rev Neurosci. 2007;8(6):451–65. - PubMed
-
- Caraiscos VB, Elliott EM, You-Ten KE, Cheng VY, Belelli D, Newell JG, Jackson MF, Lambert JJ, Rosahl TW, Wafford KA. Tonic inhibition in mouse hippocampal CA1 pyramidal neurons is mediated by a5 subunit-containing g-aminobutyric acid type A receptors. Proc Natl Acad Sci U S A. 2004;101(10):3662–7. and others. - PMC - PubMed
Publication types
MeSH terms
Substances
Grants and funding
LinkOut - more resources
Full Text Sources
Miscellaneous