Eps8 regulates axonal filopodia in hippocampal neurons in response to brain-derived neurotrophic factor (BDNF)
- PMID: 19564905
- PMCID: PMC2696597
- DOI: 10.1371/journal.pbio.1000138
Eps8 regulates axonal filopodia in hippocampal neurons in response to brain-derived neurotrophic factor (BDNF)
Erratum in
-
Correction: eps8 regulates axonal filopodia in hippocampal neurons in response to brain-derived neurotrophic factor (BDNF).PLoS Biol. 2015 Jun 3;13(6):e1002184. doi: 10.1371/journal.pbio.1002184. eCollection 2015 Jun. PLoS Biol. 2015. PMID: 26039045 Free PMC article. No abstract available.
Abstract
The regulation of filopodia plays a crucial role during neuronal development and synaptogenesis. Axonal filopodia, which are known to originate presynaptic specializations, are regulated in response to neurotrophic factors. The structural components of filopodia are actin filaments, whose dynamics and organization are controlled by ensembles of actin-binding proteins. How neurotrophic factors regulate these latter proteins remains, however, poorly defined. Here, using a combination of mouse genetic, biochemical, and cell biological assays, we show that genetic removal of Eps8, an actin-binding and regulatory protein enriched in the growth cones and developing processes of neurons, significantly augments the number and density of vasodilator-stimulated phosphoprotein (VASP)-dependent axonal filopodia. The reintroduction of Eps8 wild type (WT), but not an Eps8 capping-defective mutant, into primary hippocampal neurons restored axonal filopodia to WT levels. We further show that the actin barbed-end capping activity of Eps8 is inhibited by brain-derived neurotrophic factor (BDNF) treatment through MAPK-dependent phosphorylation of Eps8 residues S624 and T628. Additionally, an Eps8 mutant, impaired in the MAPK target sites (S624A/T628A), displays increased association to actin-rich structures, is resistant to BDNF-mediated release from microfilaments, and inhibits BDNF-induced filopodia. The opposite is observed for a phosphomimetic Eps8 (S624E/T628E) mutant. Thus, collectively, our data identify Eps8 as a critical capping protein in the regulation of axonal filopodia and delineate a molecular pathway by which BDNF, through MAPK-dependent phosphorylation of Eps8, stimulates axonal filopodia formation, a process with crucial impacts on neuronal development and synapse formation.
Conflict of interest statement
The authors have declared that no competing interests exist.
Figures
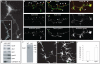
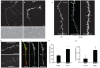
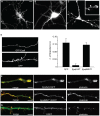
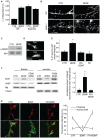
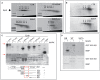
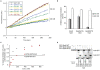
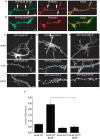
Comment in
-
The finer points of filopodia.PLoS Biol. 2009 Jun 30;7(6):e1000142. doi: 10.1371/journal.pbio.1000142. Epub 2009 Jun 30. PLoS Biol. 2009. PMID: 19564901 Free PMC article.
Similar articles
-
The finer points of filopodia.PLoS Biol. 2009 Jun 30;7(6):e1000142. doi: 10.1371/journal.pbio.1000142. Epub 2009 Jun 30. PLoS Biol. 2009. PMID: 19564901 Free PMC article.
-
The Eps8/IRSp53/VASP network differentially controls actin capping and bundling in filopodia formation.PLoS Comput Biol. 2011 Jul;7(7):e1002088. doi: 10.1371/journal.pcbi.1002088. Epub 2011 Jul 21. PLoS Comput Biol. 2011. PMID: 21814501 Free PMC article.
-
Brain-derived neurotrophic factor and epidermal growth factor activate neuronal m-calpain via mitogen-activated protein kinase-dependent phosphorylation.J Neurosci. 2010 Jan 20;30(3):1086-95. doi: 10.1523/JNEUROSCI.5120-09.2010. J Neurosci. 2010. PMID: 20089917 Free PMC article.
-
Proteomes, kinases and signalling pathways in virus-induced filopodia, as potential antiviral therapeutics targets.Rev Med Virol. 2021 Sep;31(5):1-9. doi: 10.1002/rmv.2202. Epub 2020 Dec 12. Rev Med Virol. 2021. PMID: 33314425 Free PMC article. Review.
-
Neuronal filopodia: From stochastic dynamics to robustness of brain morphogenesis.Semin Cell Dev Biol. 2023 Jan 15;133:10-19. doi: 10.1016/j.semcdb.2022.03.038. Epub 2022 Apr 6. Semin Cell Dev Biol. 2023. PMID: 35397971 Review.
Cited by
-
Erk regulation of actin capping and bundling by Eps8 promotes cortex tension and leader bleb-based migration.Elife. 2015 Jul 11;4:e08314. doi: 10.7554/eLife.08314. Elife. 2015. PMID: 26163656 Free PMC article.
-
Lack of the Actin Capping Protein, Eps8, Affects NMDA-Type Glutamate Receptor Function and Composition.Front Mol Neurosci. 2018 Sep 5;11:313. doi: 10.3389/fnmol.2018.00313. eCollection 2018. Front Mol Neurosci. 2018. PMID: 30233314 Free PMC article.
-
Gene expression profile associated with Asmt knockout-induced depression-like behaviors and exercise effects in mouse hypothalamus.Biosci Rep. 2022 Jul 29;42(7):BSR20220800. doi: 10.1042/BSR20220800. Biosci Rep. 2022. PMID: 35771226 Free PMC article.
-
The actin-binding protein EPS8 binds VE-cadherin and modulates YAP localization and signaling.J Cell Biol. 2015 Dec 21;211(6):1177-92. doi: 10.1083/jcb.201501089. Epub 2015 Dec 14. J Cell Biol. 2015. PMID: 26668327 Free PMC article.
-
Peering into tunneling nanotubes-The path forward.EMBO J. 2021 Apr 15;40(8):e105789. doi: 10.15252/embj.2020105789. Epub 2021 Mar 1. EMBO J. 2021. PMID: 33646572 Free PMC article. Review.
References
-
- Cingolani LA, Goda Y. Actin in action: the interplay between the actin cytoskeleton and synaptic efficacy. Nat Rev Neurosci. 2008;9:344–356. - PubMed
-
- Jontes JD, Buchanan J, Smith SJ. Growth cone and dendrite dynamics in zebrafish embryos: early events in synaptogenesis imaged in vivo. Nat Neurosci. 2000;3:231–237. - PubMed
-
- Toni N, Buchs PA, Nikonenko I, Bron CR, Muller D. LTP promotes formation of multiple spine synapses between a single axon terminal and a dendrite. Nature. 1999;402:421–425. - PubMed
-
- Engert F, Bonhoeffer T. Dendritic spine changes associated with hippocampal long-term synaptic plasticity. Nature. 1999;399:66–70. - PubMed
-
- Koleske AJ. Do filopodia enable the growth cone to find its way? Sci STKE. 2003;2003:pe20. - PubMed
Publication types
MeSH terms
Substances
Grants and funding
LinkOut - more resources
Full Text Sources
Molecular Biology Databases
Research Materials