Mismatch repair and nucleotide excision repair proteins cooperate in the recognition of DNA interstrand crosslinks
- PMID: 19468048
- PMCID: PMC2715249
- DOI: 10.1093/nar/gkp399
Mismatch repair and nucleotide excision repair proteins cooperate in the recognition of DNA interstrand crosslinks
Abstract
DNA interstrand crosslinks (ICLs) are among the most cytotoxic types of DNA damage, thus ICL-inducing agents such as psoralen, are clinically useful chemotherapeutics. Psoralen-modified triplex-forming oligonucleotides (TFOs) have been used to target ICLs to specific genomic sites to increase the selectivity of these agents. However, how TFO-directed psoralen ICLs (Tdp-ICLs) are recognized and processed in human cells is unclear. Previously, we reported that two essential nucleotide excision repair (NER) protein complexes, XPA-RPA and XPC-RAD23B, recognized ICLs in vitro, and that cells deficient in the DNA mismatch repair (MMR) complex MutSbeta were sensitive to psoralen ICLs. To further investigate the role of MutSbeta in ICL repair and the potential interaction between proteins from the MMR and NER pathways on these lesions, we performed electrophoretic mobility-shift assays and chromatin immunoprecipitation analysis of MutSbeta and NER proteins with Tdp-ICLs. We found that MutSbeta bound to Tdp-ICLs with high affinity and specificity in vitro and in vivo, and that MutSbeta interacted with XPA-RPA or XPC-RAD23B in recognizing Tdp-ICLs. These data suggest that proteins from the MMR and NER pathways interact in the recognition of ICLs, and provide a mechanistic link by which proteins from multiple repair pathways contribute to ICL repair.
Figures
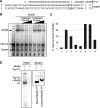
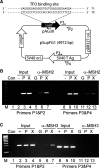
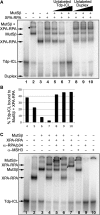
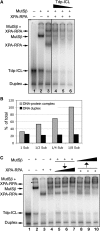
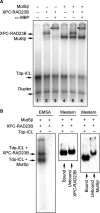
Similar articles
-
Human HMGB1 directly facilitates interactions between nucleotide excision repair proteins on triplex-directed psoralen interstrand crosslinks.DNA Repair (Amst). 2009 Jul 4;8(7):865-72. doi: 10.1016/j.dnarep.2009.04.001. Epub 2009 May 14. DNA Repair (Amst). 2009. PMID: 19446504 Free PMC article.
-
Human XPC-hHR23B interacts with XPA-RPA in the recognition of triplex-directed psoralen DNA interstrand crosslinks.Nucleic Acids Res. 2005 May 24;33(9):2993-3001. doi: 10.1093/nar/gki610. Print 2005. Nucleic Acids Res. 2005. PMID: 15914671 Free PMC article.
-
Human MLH1 protein participates in genomic damage checkpoint signaling in response to DNA interstrand crosslinks, while MSH2 functions in DNA repair.PLoS Genet. 2008 Sep 12;4(9):e1000189. doi: 10.1371/journal.pgen.1000189. PLoS Genet. 2008. PMID: 18787700 Free PMC article.
-
Targeting and processing of site-specific DNA interstrand crosslinks.Environ Mol Mutagen. 2010 Jul;51(6):527-39. doi: 10.1002/em.20557. Environ Mol Mutagen. 2010. PMID: 20196133 Free PMC article. Review.
-
Critical DNA damage recognition functions of XPC-hHR23B and XPA-RPA in nucleotide excision repair.Mol Carcinog. 2003 Sep;38(1):1-13. doi: 10.1002/mc.10143. Mol Carcinog. 2003. PMID: 12949838 Review.
Cited by
-
Dynamic alternative DNA structures in biology and disease.Nat Rev Genet. 2023 Apr;24(4):211-234. doi: 10.1038/s41576-022-00539-9. Epub 2022 Oct 31. Nat Rev Genet. 2023. PMID: 36316397 Free PMC article. Review.
-
The yin and yang of repair mechanisms in DNA structure-induced genetic instability.Mutat Res. 2013 Mar-Apr;743-744:118-131. doi: 10.1016/j.mrfmmm.2012.11.005. Epub 2012 Dec 3. Mutat Res. 2013. PMID: 23219604 Free PMC article. Review.
-
Friedreich's ataxia induced pluripotent stem cells model intergenerational GAA⋅TTC triplet repeat instability.Cell Stem Cell. 2010 Nov 5;7(5):631-7. doi: 10.1016/j.stem.2010.09.014. Cell Stem Cell. 2010. PMID: 21040903 Free PMC article.
-
MutLalpha and proliferating cell nuclear antigen share binding sites on MutSbeta.J Biol Chem. 2010 Apr 9;285(15):11730-9. doi: 10.1074/jbc.M110.104125. Epub 2010 Feb 12. J Biol Chem. 2010. PMID: 20154325 Free PMC article.
-
Progressive GAA.TTC repeat expansion in human cell lines.PLoS Genet. 2009 Oct;5(10):e1000704. doi: 10.1371/journal.pgen.1000704. Epub 2009 Oct 30. PLoS Genet. 2009. PMID: 19876374 Free PMC article.
References
-
- Dronkert ML, Kanaar R. Repair of DNA interstrand cross-links. Mutat. Res. 2001;486:217–247. - PubMed
-
- McHugh PJ, Spanswick VJ, Hartley JA. Repair of DNA interstrand crosslinks: molecular mechanisms and clinical relevance. Lancet Oncol. 2001;2:483–490. - PubMed
-
- Momtaz K, Fitzpatrick TB. The benefits and risks of long-term PUVA photochemotherapy. Dermatol. Clin. 1998;16:227–234. - PubMed
Publication types
MeSH terms
Substances
Grants and funding
LinkOut - more resources
Full Text Sources
Miscellaneous