Inactivation of Drosophila Huntingtin affects long-term adult functioning and the pathogenesis of a Huntington's disease model
- PMID: 19380309
- PMCID: PMC2675792
- DOI: 10.1242/dmm.000653
Inactivation of Drosophila Huntingtin affects long-term adult functioning and the pathogenesis of a Huntington's disease model
Abstract
A polyglutamine expansion in the huntingtin (HTT) gene causes neurodegeneration in Huntington's disease (HD), but the in vivo function of the native protein (Htt) is largely unknown. Numerous biochemical and in vitro studies have suggested a role for Htt in neuronal development, synaptic function and axonal trafficking. To test these models, we generated a null mutant in the putative Drosophila HTT homolog (htt, hereafter referred to asdhtt) and, surprisingly, found that dhtt mutant animals are viable with no obvious developmental defects. Instead, dhtt is required for maintaining the mobility and long-term survival of adult animals, and for modulating axonal terminal complexity in the adult brain. Furthermore, removing endogenous dhtt significantly accelerates the neurodegenerative phenotype associated with a Drosophila model of polyglutamine Htt toxicity (HD-Q93), providing in vivo evidence that disrupting the normal function of Htt might contribute to HD pathogenesis.
Figures
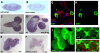
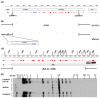
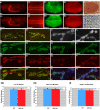
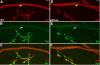
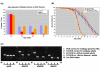
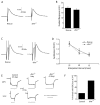
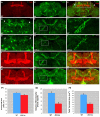
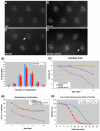
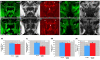
Comment in
-
Hunting for the function of Huntingtin.Dis Model Mech. 2009 May-Jun;2(5-6):199-200. doi: 10.1242/dmm.003376. Dis Model Mech. 2009. PMID: 19407322 Free PMC article. No abstract available.
Similar articles
-
Hunting for the function of Huntingtin.Dis Model Mech. 2009 May-Jun;2(5-6):199-200. doi: 10.1242/dmm.003376. Dis Model Mech. 2009. PMID: 19407322 Free PMC article. No abstract available.
-
Downregulation of glial genes involved in synaptic function mitigates Huntington's disease pathogenesis.Elife. 2021 Apr 19;10:e64564. doi: 10.7554/eLife.64564. Elife. 2021. PMID: 33871358 Free PMC article.
-
Pathogenic Huntington Alters BMP Signaling and Synaptic Growth through Local Disruptions of Endosomal Compartments.J Neurosci. 2017 Mar 22;37(12):3425-3439. doi: 10.1523/JNEUROSCI.2752-16.2017. Epub 2017 Feb 24. J Neurosci. 2017. PMID: 28235896 Free PMC article.
-
Choosing and using Drosophila models to characterize modifiers of Huntington's disease.Biochem Soc Trans. 2012 Aug;40(4):739-45. doi: 10.1042/BST20120072. Biochem Soc Trans. 2012. PMID: 22817726 Review.
-
Huntingtin and its role in neuronal degeneration.Neuroscientist. 2004 Oct;10(5):467-75. doi: 10.1177/1073858404266777. Neuroscientist. 2004. PMID: 15359012 Review.
Cited by
-
High-content chemical and RNAi screens for suppressors of neurotoxicity in a Huntington's disease model.PLoS One. 2011;6(8):e23841. doi: 10.1371/journal.pone.0023841. Epub 2011 Aug 31. PLoS One. 2011. PMID: 21909362 Free PMC article.
-
Huntington's disease: progress toward effective disease-modifying treatments and a cure.Hum Mol Genet. 2010 Apr 15;19(R1):R98-R102. doi: 10.1093/hmg/ddq148. Epub 2010 Apr 26. Hum Mol Genet. 2010. PMID: 20421366 Free PMC article. Review.
-
Hsp40 overexpression in pacemaker neurons delays circadian dysfunction in a Drosophila model of Huntington's disease.Dis Model Mech. 2022 Jun 1;15(6):dmm049447. doi: 10.1242/dmm.049447. Epub 2022 Jun 28. Dis Model Mech. 2022. PMID: 35645202 Free PMC article.
-
Delayed Induction of Human NTE (PNPLA6) Rescues Neurodegeneration and Mobility Defects of Drosophila swiss cheese (sws) Mutants.PLoS One. 2015 Dec 15;10(12):e0145356. doi: 10.1371/journal.pone.0145356. eCollection 2015. PLoS One. 2015. PMID: 26671664 Free PMC article.
-
A burst of ABC genes in the genome of the polyphagous spider mite Tetranychus urticae.BMC Genomics. 2013 May 10;14:317. doi: 10.1186/1471-2164-14-317. BMC Genomics. 2013. PMID: 23663308 Free PMC article.
References
-
- Andrade M.A., Bork P. (1995). HEAT repeats in the Huntington’s disease protein. Nat Genet. 11, 115–116 - PubMed
-
- Andrade M.A., Petosa C., O’Donoghue S.I., Muller C.W., Bork P. (2001). Comparison of ARM and HEAT protein repeats. J Mol Biol. 309, 1–18 - PubMed
-
- Atkinson N.S., Robertson G.A., Ganetzky B. (1991). A component of calcium-activated potassium channels encoded by the Drosophila slo locus. Science 253, 551–555 - PubMed
-
- Auerbach W., Hurlbert M.S., Hilditch-Maguire P., Wadghiri Y.Z., Wheeler V.C., Cohen S.I., Joyner A.L., MacDonald M.E., Turnbull D.H. (2001). The HD mutation causes progressive lethal neurological disease in mice expressing reduced levels of huntingtin. Hum Mol Genet. 10, 2515–2523 - PubMed
-
- Budnik V., Gramates L.S. (1999). Neuromuscular Junctions in Drosophila. San Diego, CA: London: Academic Press
Publication types
MeSH terms
Substances
Grants and funding
LinkOut - more resources
Full Text Sources
Medical
Molecular Biology Databases
Research Materials
Miscellaneous