Fidelity at the molecular level: lessons from protein synthesis
- PMID: 19239893
- PMCID: PMC3691815
- DOI: 10.1016/j.cell.2009.01.036
Fidelity at the molecular level: lessons from protein synthesis
Abstract
The faithful and rapid translation of genetic information into peptide sequences is an indispensable property of the ribosome. The mechanistic understanding of strategies used by the ribosome to achieve both speed and fidelity during translation results from nearly a half century of biochemical and structural studies. Emerging from these studies is the common theme that the ribosome uses local as well as remote conformational switches to govern induced-fit mechanisms that ensure accuracy in codon recognition during both tRNA selection and translation termination.
Figures
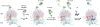
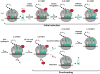
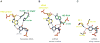
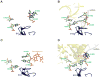
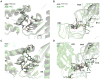
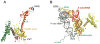
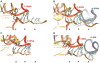
Similar articles
-
Quality control of mRNA decoding on the bacterial ribosome.Adv Protein Chem Struct Biol. 2012;86:95-128. doi: 10.1016/B978-0-12-386497-0.00003-7. Adv Protein Chem Struct Biol. 2012. PMID: 22243582 Review.
-
Polyamines accelerate codon recognition by transfer RNAs on the ribosome.Biochemistry. 2010 Aug 24;49(33):7179-89. doi: 10.1021/bi1009776. Biochemistry. 2010. PMID: 20666453 Free PMC article.
-
Quality control by the ribosome following peptide bond formation.Nature. 2009 Jan 8;457(7226):161-6. doi: 10.1038/nature07582. Epub 2008 Dec 17. Nature. 2009. PMID: 19092806 Free PMC article.
-
Altered tRNA dynamics during translocation on slippery mRNA as determinant of spontaneous ribosome frameshifting.Nat Commun. 2022 Jul 22;13(1):4231. doi: 10.1038/s41467-022-31852-w. Nat Commun. 2022. PMID: 35869111 Free PMC article.
-
[tRNA-binding centers of Escherichia coli ribosomes and their structural organization].Mol Biol (Mosk). 1984 Sep-Oct;18(5):1194-207. Mol Biol (Mosk). 1984. PMID: 6209546 Review. Russian.
Cited by
-
Knockout of AtMKK1 enhances salt tolerance and modifies metabolic activities in Arabidopsis.Plant Signal Behav. 2013 May;8(5):e24206. doi: 10.4161/psb.24206. Epub 2013 Mar 19. Plant Signal Behav. 2013. PMID: 23511202 Free PMC article.
-
Yeast Trm7 interacts with distinct proteins for critical modifications of the tRNAPhe anticodon loop.RNA. 2012 Oct;18(10):1921-33. doi: 10.1261/rna.035287.112. Epub 2012 Aug 21. RNA. 2012. PMID: 22912484 Free PMC article.
-
Estimating selection on synonymous codon usage from noisy experimental data.Mol Biol Evol. 2013 Jun;30(6):1438-53. doi: 10.1093/molbev/mst051. Epub 2013 Mar 14. Mol Biol Evol. 2013. PMID: 23493257 Free PMC article.
-
Insights into the base-pairing preferences of 8-oxoguanosine on the ribosome.Nucleic Acids Res. 2019 Oct 10;47(18):9857-9870. doi: 10.1093/nar/gkz701. Nucleic Acids Res. 2019. PMID: 31400119 Free PMC article.
-
Complex molecular dynamics in the spotlight.Nat Biotechnol. 2010 Jun;28(6):564-5. doi: 10.1038/nbt0610-564. Nat Biotechnol. 2010. PMID: 20531335 No abstract available.
References
-
- Ban N, Nissen P, Hansen J, Moore PB, Steitz TA. The complete atomic structure of the large ribosomal subunit at 2.4 Å resolution. Science. 2000;289:905–920. - PubMed
-
- Bilgin N, Claesens F, Pahverk H, Ehrenberg M. Kinetic properties of Escherichia coli ribosomes with altered forms of S12. J Mol Biol. 1992;224:1011–1027. - PubMed
-
- Blanchard SC, Gonzalez RL, Kim HD, Chu S, Puglisi JD. tRNA selection and kinetic proofreading in translation. Nat Struct Mol Biol. 2004a;11:1008–1014. - PubMed
Publication types
MeSH terms
Substances
Grants and funding
LinkOut - more resources
Full Text Sources
Other Literature Sources