Diastolic dysfunction in familial hypertrophic cardiomyopathy transgenic model mice
- PMID: 19150977
- PMCID: PMC2721639
- DOI: 10.1093/cvr/cvp016
Diastolic dysfunction in familial hypertrophic cardiomyopathy transgenic model mice
Abstract
Aims: Several mutations in the ventricular myosin regulatory light chain (RLC) were identified to cause familial hypertrophic cardiomyopathy (FHC). Based on our previous cellular findings showing delayed calcium transients in electrically stimulated intact papillary muscle fibres from transgenic Tg-R58Q and Tg-N47K mice and, in addition, prolonged force transients in Tg-R58Q fibres, we hypothesized that the malignant FHC phenotype associated with the R58Q mutation is most likely related to diastolic dysfunction.
Methods and results: Cardiac morphology and in vivo haemodynamics by echocardiography as well as cardiac function in isolated perfused working hearts were assessed in transgenic (Tg) mutant mice. The ATPase-pCa relationship was determined in myofibrils isolated from Tg mouse hearts. In addition, the effect of both mutations on RLC phosphorylation was examined in rapidly frozen ventricular samples from Tg mice. Significantly, decreased cardiac function was observed in isolated perfused working hearts from both Tg-R58Q and Tg-N47K mice. However, echocardiographic examination showed significant alterations in diastolic transmitral velocities and deceleration time only in Tg-R58Q myocardium. Likewise, changes in Ca(2+) sensitivity, cooperativity, and an elevated level of ATPase activity at low [Ca(2+)] were only observed in myofibrils from Tg-R58Q mice. In addition, the R58Q mutation and not the N47K led to reduced RLC phosphorylation in Tg ventricles.
Conclusion: Our results suggest that the N47K and R58Q mutations may act through similar mechanisms, leading to compensatory hypertrophy of the functionally compromised myocardium, but the malignant R58Q phenotype is most likely associated with more severe alterations in cardiac performance manifested as impaired relaxation and global diastolic dysfunction. At the molecular level, we suggest that by reducing the phosphorylation of RLC, the R58Q mutation decreases the kinetics of myosin cross-bridges, leading to an increased myofilament calcium sensitivity and to overall changes in intracellular Ca(2+) homeostasis.
Figures

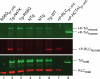
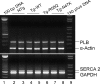
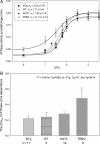
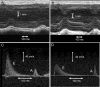
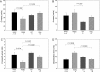
Similar articles
-
Cross-bridge kinetics in myofibrils containing familial hypertrophic cardiomyopathy R58Q mutation in the regulatory light chain of myosin.J Theor Biol. 2011 Sep 7;284(1):71-81. doi: 10.1016/j.jtbi.2011.06.014. Epub 2011 Jun 24. J Theor Biol. 2011. PMID: 21723297 Free PMC article.
-
Prolonged Ca2+ and force transients in myosin RLC transgenic mouse fibers expressing malignant and benign FHC mutations.J Mol Biol. 2006 Aug 11;361(2):286-99. doi: 10.1016/j.jmb.2006.06.018. Epub 2006 Jun 27. J Mol Biol. 2006. PMID: 16837010
-
Discrete effects of A57G-myosin essential light chain mutation associated with familial hypertrophic cardiomyopathy.Am J Physiol Heart Circ Physiol. 2013 Aug 15;305(4):H575-89. doi: 10.1152/ajpheart.00107.2013. Epub 2013 Jun 7. Am J Physiol Heart Circ Physiol. 2013. PMID: 23748425 Free PMC article.
-
Cellular and molecular aspects of familial hypertrophic cardiomyopathy caused by mutations in the cardiac troponin I gene.Mol Cell Biochem. 2004 Aug;263(1-2):99-114. doi: 10.1023/B:MCBI.0000041852.42291.aa. Mol Cell Biochem. 2004. PMID: 15524171 Review.
-
Molecular mechanisms of cardiomyopathy phenotypes associated with myosin light chain mutations.J Muscle Res Cell Motil. 2015 Dec;36(6):433-45. doi: 10.1007/s10974-015-9423-3. Epub 2015 Sep 18. J Muscle Res Cell Motil. 2015. PMID: 26385864 Free PMC article. Review.
Cited by
-
Cardiac-specific over-expression of epidermal growth factor receptor 2 (ErbB2) induces pro-survival pathways and hypertrophic cardiomyopathy in mice.PLoS One. 2012;7(8):e42805. doi: 10.1371/journal.pone.0042805. Epub 2012 Aug 9. PLoS One. 2012. PMID: 22912742 Free PMC article.
-
Gene expression patterns in transgenic mouse models of hypertrophic cardiomyopathy caused by mutations in myosin regulatory light chain.Arch Biochem Biophys. 2016 Jul 1;601:121-32. doi: 10.1016/j.abb.2016.02.022. Epub 2016 Feb 22. Arch Biochem Biophys. 2016. PMID: 26906074 Free PMC article.
-
To lie or not to lie: Super-relaxing with myosins.Elife. 2021 Feb 10;10:e63703. doi: 10.7554/eLife.63703. Elife. 2021. PMID: 33565963 Free PMC article.
-
Cardiac Sarcomere Signaling in Health and Disease.Int J Mol Sci. 2022 Dec 19;23(24):16223. doi: 10.3390/ijms232416223. Int J Mol Sci. 2022. PMID: 36555864 Free PMC article. Review.
-
Cross-bridge kinetics in myofibrils containing familial hypertrophic cardiomyopathy R58Q mutation in the regulatory light chain of myosin.J Theor Biol. 2011 Sep 7;284(1):71-81. doi: 10.1016/j.jtbi.2011.06.014. Epub 2011 Jun 24. J Theor Biol. 2011. PMID: 21723297 Free PMC article.
References
-
- Maron BJ. Hypertrophic cardiomyopathy: a systematic review. JAMA. 2002;287:1308–1320. - PubMed
-
- Seidman CE, Seidman JG. Molecular genetic studies of familial hypertrophic cardiomyopathy. Basic Res Cardiol. 1998;93:13–16. - PubMed
-
- Tardiff J. Sarcomeric proteins and familial hypertrophic cardiomyopathy: linking mutations in structural proteins to complex cardiovascular phenotypes. Heart Fail Rev. 2005;10:237–248. - PubMed
-
- Alcalai R, Seidman JG, Seidman CE. Genetic basis of hypertrophic cardiomyopathy: from bench to the clinics. J Cardiovasc Electrophysiol. 2008;19:104–110. - PubMed
-
- Szczesna D. Regulatory light chains of striated muscle myosin. Structure, function and malfunction. Curr Drug Targets Cardiovasc Haematol Disord. 2003;3:187–197. - PubMed
Publication types
MeSH terms
Substances
Grants and funding
LinkOut - more resources
Full Text Sources
Molecular Biology Databases
Miscellaneous