Translation elongation factor 1A is a component of the tombusvirus replicase complex and affects the stability of the p33 replication co-factor
- PMID: 19131084
- PMCID: PMC2785496
- DOI: 10.1016/j.virol.2008.11.041
Translation elongation factor 1A is a component of the tombusvirus replicase complex and affects the stability of the p33 replication co-factor
Abstract
Host RNA-binding proteins are likely to play multiple, integral roles during replication of plus-strand RNA viruses. To identify host proteins that bind to viral RNAs, we took a global approach based on the yeast proteome microarray, which contains 4080 purified yeast proteins. The biotin-labeled RNA probes included two distantly related RNA viruses, namely Tomato bushy stunt virus (TBSV) and Brome mosaic virus (BMV). Altogether, we have identified 57 yeast proteins that bound to TBSV RNA and/or BMV RNA. Among the identified host proteins, eleven bound to TBSV RNA and seven bound to BMV RNA with high selectivity, whereas the remaining 39 host proteins bound to both viral RNAs. The interaction between the TBSV replicon RNA and five of the identified host proteins was confirmed via gel-mobility shift and co-purification experiments from yeast. Over-expression of the host proteins in yeast, a model host for TBSV, revealed 4 host proteins that enhanced TBSV replication as well as 14 proteins that inhibited replication. Detailed analysis of one of the identified yeast proteins binding to TBSV RNA, namely translation elongation factor eEF1A, revealed that it is present in the highly purified tombusvirus replicase complex. We also demonstrate binding of eEF1A to the p33 replication protein and a known cis-acting element at the 3' end of TBSV RNA. Using a functional mutant of eEF1A, we provide evidence on the involvement of eEF1A in TBSV replication.
Figures
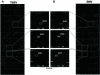
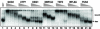
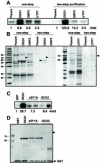
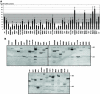
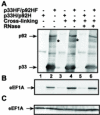
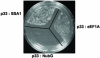
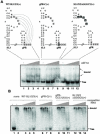
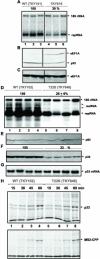
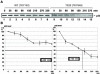
Similar articles
-
Cdc34p ubiquitin-conjugating enzyme is a component of the tombusvirus replicase complex and ubiquitinates p33 replication protein.J Virol. 2008 Jul;82(14):6911-26. doi: 10.1128/JVI.00702-08. Epub 2008 May 7. J Virol. 2008. PMID: 18463149 Free PMC article.
-
Reconstitution of an RNA Virus Replicase in Artificial Giant Unilamellar Vesicles Supports Full Replication and Provides Protection for the Double-Stranded RNA Replication Intermediate.J Virol. 2020 Aug 31;94(18):e00267-20. doi: 10.1128/JVI.00267-20. Print 2020 Aug 31. J Virol. 2020. PMID: 32641477 Free PMC article.
-
Methylation of translation elongation factor 1A by the METTL10-like See1 methyltransferase facilitates tombusvirus replication in yeast and plants.Virology. 2014 Jan 5;448:43-54. doi: 10.1016/j.virol.2013.09.012. Epub 2013 Oct 18. Virology. 2014. PMID: 24314635
-
Tombusvirus polymerase: Structure and function.Virus Res. 2017 Apr 15;234:74-86. doi: 10.1016/j.virusres.2017.01.012. Epub 2017 Jan 19. Virus Res. 2017. PMID: 28111194 Review.
-
Global genomics and proteomics approaches to identify host factors as targets to induce resistance against Tomato bushy stunt virus.Adv Virus Res. 2010;76:123-77. doi: 10.1016/S0065-3527(10)76004-8. Epub 2010 Mar 31. Adv Virus Res. 2010. PMID: 20965073 Free PMC article. Review.
Cited by
-
Cotton leaf curl Multan virus differentially regulates innate antiviral immunity of whitefly (Bemisia tabaci) vector to promote cryptic species-dependent virus acquisition.Front Plant Sci. 2022 Nov 14;13:1040547. doi: 10.3389/fpls.2022.1040547. eCollection 2022. Front Plant Sci. 2022. PMID: 36452094 Free PMC article.
-
Conserved motifs in a tombusvirus polymerase modulate genome replication, subgenomic transcription, and amplification of defective interfering RNAs.J Virol. 2015 Mar;89(6):3236-46. doi: 10.1128/JVI.03378-14. Epub 2015 Jan 7. J Virol. 2015. PMID: 25568204 Free PMC article.
-
Synergistic roles of eukaryotic translation elongation factors 1Bγ and 1A in stimulation of tombusvirus minus-strand synthesis.PLoS Pathog. 2011 Dec;7(12):e1002438. doi: 10.1371/journal.ppat.1002438. Epub 2011 Dec 15. PLoS Pathog. 2011. PMID: 22194687 Free PMC article.
-
A host RNA helicase-like protein, AtRH8, interacts with the potyviral genome-linked protein, VPg, associates with the virus accumulation complex, and is essential for infection.Plant Physiol. 2010 Jan;152(1):255-66. doi: 10.1104/pp.109.147983. Epub 2009 Oct 30. Plant Physiol. 2010. PMID: 19880609 Free PMC article.
-
A temperature sensitive mutant of heat shock protein 70 reveals an essential role during the early steps of tombusvirus replication.Virology. 2009 Nov 10;394(1):28-38. doi: 10.1016/j.virol.2009.08.003. Epub 2009 Sep 12. Virology. 2009. PMID: 19748649 Free PMC article.
References
-
- Anand M, Balar B, Ulloque R, Gross SR, Kinzy TG. Domain and nucleotide dependence of the interaction between Saccharomyces cerevisiae translation elongation factors 3 and 1A. J Biol Chem. 2006;281(43):32318–26. - PubMed
Publication types
MeSH terms
Substances
Grants and funding
LinkOut - more resources
Full Text Sources
Miscellaneous