Contrasting the functional properties of GABAergic axon terminals with single and multiple synapses in the thalamus
- PMID: 19005050
- PMCID: PMC6671651
- DOI: 10.1523/JNEUROSCI.3183-08.2008
Contrasting the functional properties of GABAergic axon terminals with single and multiple synapses in the thalamus
Abstract
Diverse sources of GABAergic inhibition are a major feature of cortical networks, but distinct inhibitory input systems have not been systematically characterized in the thalamus. Here, we contrasted the properties of two independent GABAergic pathways in the posterior thalamic nucleus of rat, one input from the reticular thalamic nucleus (nRT), and one "extrareticular" input from the anterior pretectal nucleus (APT). The vast majority of nRT-thalamic terminals formed single synapses per postsynaptic target and innervated thin distal dendrites of relay cells. In contrast, single APT-thalamic terminals formed synaptic contacts exclusively via multiple, closely spaced synapses on thick relay cell dendrites. Quantal analysis demonstrated that the two inputs displayed comparable quantal amplitudes, release probabilities, and multiple release sites. The morphological and physiological data together indicated multiple, single-site contacts for nRT and multisite contacts for APT axons. The contrasting synaptic arrangements of the two pathways were paralleled by different short-term plasticities. The multisite APT-thalamic pathway showed larger charge transfer during 50-100 Hz stimulation compared with the nRT pathway and a greater persistent inhibition accruing during stimulation trains. Our results demonstrate that the two inhibitory systems are morpho-functionally distinct and suggest and that multisite GABAergic terminals are tailored for maintained synaptic inhibition even at high presynaptic firing rates. These data explain the efficacy of extrareticular inhibition in timing relay cell activity in sensory and motor thalamic nuclei. Finally, based on the classic nomenclature and the difference between reticular and extrareticular terminals, we define a novel, multisite GABAergic terminal type (F3) in the thalamus.
Figures
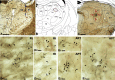
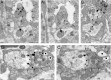
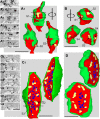
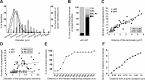
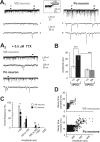
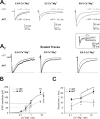
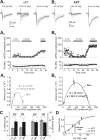
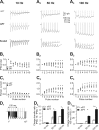
Similar articles
-
Synaptic organization of GABAergic projections from the substantia nigra pars reticulata and the reticular thalamic nucleus to the parafascicular thalamic nucleus in the rat.Brain Res. 2002 Dec 13;957(2):231-41. doi: 10.1016/s0006-8993(02)03554-0. Brain Res. 2002. PMID: 12445965
-
Selective GABAergic control of higher-order thalamic relays.Neuron. 2005 Mar 24;45(6):929-40. doi: 10.1016/j.neuron.2005.01.048. Neuron. 2005. PMID: 15797553
-
Selective GABAergic innervation of thalamic nuclei from zona incerta.Eur J Neurosci. 2002 Sep;16(6):999-1014. doi: 10.1046/j.1460-9568.2002.02157.x. Eur J Neurosci. 2002. PMID: 12383229
-
Thalamic organization and function after Cajal.Prog Brain Res. 2002;136:333-57. doi: 10.1016/s0079-6123(02)36029-1. Prog Brain Res. 2002. PMID: 12143393 Review.
-
The vibrissal system as a model of thalamic operations.Prog Brain Res. 2005;149:31-40. doi: 10.1016/S0079-6123(05)49003-2. Prog Brain Res. 2005. PMID: 16226574 Review.
Cited by
-
Thalamic Inhibition: Diverse Sources, Diverse Scales.Trends Neurosci. 2016 Oct;39(10):680-693. doi: 10.1016/j.tins.2016.08.001. Epub 2016 Aug 30. Trends Neurosci. 2016. PMID: 27589879 Free PMC article. Review.
-
Structural Plasticity of GABAergic Pallidothalamic Terminals in MPTP-Treated Parkinsonian Monkeys: A 3D Electron Microscopic Analysis.eNeuro. 2024 Mar 21;11(3):ENEURO.0241-23.2024. doi: 10.1523/ENEURO.0241-23.2024. Print 2024 Mar. eNeuro. 2024. PMID: 38514185 Free PMC article.
-
The locus coeruleus drives disinhibition in the midline thalamus via a dopaminergic mechanism.Nat Neurosci. 2018 Jul;21(7):963-973. doi: 10.1038/s41593-018-0167-4. Epub 2018 Jun 18. Nat Neurosci. 2018. PMID: 29915192 Free PMC article.
-
Phasic, nonsynaptic GABA-A receptor-mediated inhibition entrains thalamocortical oscillations.J Neurosci. 2014 May 21;34(21):7137-47. doi: 10.1523/JNEUROSCI.4386-13.2014. J Neurosci. 2014. PMID: 24849349 Free PMC article.
-
Open-loop organization of thalamic reticular nucleus and dorsal thalamus: a computational model.J Neurophysiol. 2015 Oct;114(4):2353-67. doi: 10.1152/jn.00926.2014. Epub 2015 Aug 19. J Neurophysiol. 2015. PMID: 26289472 Free PMC article.
References
-
- Barthó P, Freund TF, Acsády L. Selective GABAergic innervation of thalamic nuclei from zona incerta. Eur J Neurosci. 2002;16:999–1014. - PubMed
-
- Berod A, Hartman BK, Pujol JF. Importance of fixation in immunohistochemistry: use of formaldehyde solutions at variable pH for the localization of tyrosine hydroxylase. J Histochem Cytochem. 1981;29:844–850. - PubMed
Publication types
MeSH terms
Substances
Grants and funding
LinkOut - more resources
Full Text Sources
Miscellaneous