Drosophila bloom helicase maintains genome integrity by inhibiting recombination between divergent DNA sequences
- PMID: 18978019
- PMCID: PMC2588521
- DOI: 10.1093/nar/gkn793
Drosophila bloom helicase maintains genome integrity by inhibiting recombination between divergent DNA sequences
Abstract
DNA double strand breaks (DSB) can be repaired either via a sequence independent joining of DNA ends or via homologous recombination. We established a detection system in Drosophila melanogaster to investigate the impact of sequence constraints on the usage of the homology based DSB repair via single strand annealing (SSA), which leads to recombination between direct repeats with concomitant loss of one repeat copy. First of all, we find the SSA frequency to be inversely proportional to the spacer length between the repeats, for spacers up to 2.4 kb in length. We further show that SSA between divergent repeats (homeologous SSA) is suppressed in cell cultures and in vivo in a sensitive manner, recognizing sequence divergences smaller than 0.5%. Finally, we demonstrate that the suppression of homeologous SSA depends on the Bloom helicase (Blm), encoded by the Drosophila gene mus309. Suppression of homeologous recombination is a novel function of Blm in ensuring genomic integrity, not described to date in mammalian systems. Unexpectedly, distinct from its function in Saccharomyces cerevisiae, the mismatch repair factor Msh2 encoded by spel1 does not suppress homeologous SSA in Drosophila.
Figures
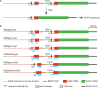
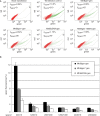
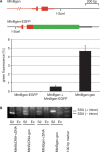
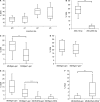
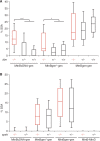
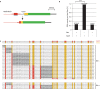
Similar articles
-
The Role of Blm Helicase in Homologous Recombination, Gene Conversion Tract Length, and Recombination Between Diverged Sequences in Drosophilamelanogaster.Genetics. 2017 Nov;207(3):923-933. doi: 10.1534/genetics.117.300285. Epub 2017 Sep 14. Genetics. 2017. PMID: 28912341 Free PMC article.
-
The Role of Drosophila CtIP in Homology-Directed Repair of DNA Double-Strand Breaks.Genes (Basel). 2021 Sep 16;12(9):1430. doi: 10.3390/genes12091430. Genes (Basel). 2021. PMID: 34573412 Free PMC article.
-
Biochemical Activities and Genetic Functions of the Drosophila melanogaster Fancm Helicase in DNA Repair.Genetics. 2016 Oct;204(2):531-541. doi: 10.1534/genetics.116.192534. Epub 2016 Jul 27. Genetics. 2016. PMID: 27466228 Free PMC article.
-
Ionizing radiation and genetic risks XIV. Potential research directions in the post-genome era based on knowledge of repair of radiation-induced DNA double-strand breaks in mammalian somatic cells and the origin of deletions associated with human genomic disorders.Mutat Res. 2005 Oct 15;578(1-2):333-70. doi: 10.1016/j.mrfmmm.2005.06.020. Epub 2005 Aug 5. Mutat Res. 2005. PMID: 16084534 Review.
-
Regulation of Single-Strand Annealing and its Role in Genome Maintenance.Trends Genet. 2016 Sep;32(9):566-575. doi: 10.1016/j.tig.2016.06.007. Epub 2016 Jul 19. Trends Genet. 2016. PMID: 27450436 Free PMC article. Review.
Cited by
-
Age- and temperature-dependent somatic mutation accumulation in Drosophila melanogaster.PLoS Genet. 2010 May 13;6(5):e1000950. doi: 10.1371/journal.pgen.1000950. PLoS Genet. 2010. PMID: 20485564 Free PMC article.
-
DNA mismatch repair system: repercussions in cellular homeostasis and relationship with aging.Oxid Med Cell Longev. 2012;2012:728430. doi: 10.1155/2012/728430. Epub 2012 Nov 8. Oxid Med Cell Longev. 2012. PMID: 23213348 Free PMC article. Review.
-
A new powerful method for site-specific transgene stabilization based on chromosomal double-strand break repair.PLoS One. 2011;6(10):e26422. doi: 10.1371/journal.pone.0026422. Epub 2011 Oct 17. PLoS One. 2011. PMID: 22022613 Free PMC article.
-
The Role of Blm Helicase in Homologous Recombination, Gene Conversion Tract Length, and Recombination Between Diverged Sequences in Drosophilamelanogaster.Genetics. 2017 Nov;207(3):923-933. doi: 10.1534/genetics.117.300285. Epub 2017 Sep 14. Genetics. 2017. PMID: 28912341 Free PMC article.
-
Loss of the bloom syndrome helicase increases DNA ligase 4-independent genome rearrangements and tumorigenesis in aging Drosophila.Genome Biol. 2011 Dec 19;12(12):R121. doi: 10.1186/gb-2011-12-12-r121. Genome Biol. 2011. PMID: 22183041 Free PMC article.
References
-
- Shrivastav M, De Haro LP, Nickoloff JA. Regulation of DNA double-strand break repair pathway choice. Cell Res. 2008;18:134–147. - PubMed
-
- Pastink A, Eeken JC, Lohman PH. Genomic integrity and the repair of double-strand DNA breaks. Mutat. Res. 2001;480:37–50. - PubMed
-
- Gonda DK, Radding CM. By searching processively RecA protein pairs DNA molecules that share a limited stretch of homology. Cell. 1983;34:647–654. - PubMed
Publication types
MeSH terms
Substances
LinkOut - more resources
Full Text Sources
Molecular Biology Databases
Research Materials