Structural and thermodynamic characterization of T4 lysozyme mutants and the contribution of internal cavities to pressure denaturation
- PMID: 18816066
- PMCID: PMC2664309
- DOI: 10.1021/bi801287m
Structural and thermodynamic characterization of T4 lysozyme mutants and the contribution of internal cavities to pressure denaturation
Abstract
Using small-angle X-ray scattering (SAXS) and tryptophan fluorescence spectroscopy, we have identified multiple compact denatured states of a series of T4 lysozyme mutants that are stabilized by high pressures. Recent studies imply that the mechanism of pressure denaturation is the penetration of water into the protein rather than the transfer of hydrophobic residues into water. To investigate water penetration and the volume change associated with pressure denaturation, we studied the solution behavior of four T4 lysozyme mutants having different cavity volumes at low and neutral pH up to a pressure of 400 MPa (0.1 MPa = 0.9869 atm). At low pH, L99A T4 lysozyme expanded from a compact folded state to a partially unfolded state with a corresponding change in radius of gyration from 17 to 32 A. The volume change upon denaturation correlated well with the total cavity volume, indicating that all of the molecule's major cavities are hydrated with pressure. As a direct comparison to high-pressure crystal structures of L99A T4 lysozyme solved at neutral pH [Collins, M. D., Hummer, G., Quillin, M. L., Matthews, B. W., and Gruner, S. M. (2005) Proc. Natl. Acad. Sci. U.S.A. 102, 16668-16671], pressure denaturation of L99A and the structurally similar L99G/E108V mutant was studied at neutral pH. The pressure-denatured state at neutral pH is even more compact than at low pH, and the small volume changes associated with denaturation suggest that the preferential filling of large cavities is responsible for the compactness of the pressure-denatured state. These results confirm that pressure denaturation is characteristically distinct from thermal or chemical denaturation.
Figures
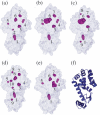
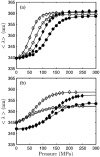
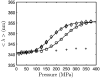
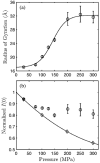
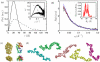
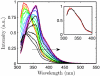
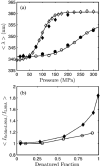
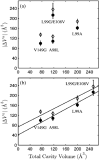
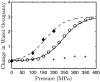
Similar articles
-
Structure-relaxation mechanism for the response of T4 lysozyme cavity mutants to hydrostatic pressure.Proc Natl Acad Sci U S A. 2015 May 12;112(19):E2437-46. doi: 10.1073/pnas.1506505112. Epub 2015 Apr 27. Proc Natl Acad Sci U S A. 2015. PMID: 25918400 Free PMC article.
-
Structural analysis of a non-contiguous second-site revertant in T4 lysozyme shows that increasing the rigidity of a protein can enhance its stability.J Mol Biol. 1999 Oct 8;292(5):1111-20. doi: 10.1006/jmbi.1999.3102. J Mol Biol. 1999. PMID: 10512706
-
Structural and energetic responses to cavity-creating mutations in hydrophobic cores: observation of a buried water molecule and the hydrophilic nature of such hydrophobic cavities.Biochemistry. 1996 Apr 9;35(14):4298-305. doi: 10.1021/bi9524676. Biochemistry. 1996. PMID: 8605178
-
Structural and genetic analysis of the folding and function of T4 lysozyme.FASEB J. 1996 Jan;10(1):35-41. doi: 10.1096/fasebj.10.1.8566545. FASEB J. 1996. PMID: 8566545 Review.
-
Lessons from the lysozyme of phage T4.Protein Sci. 2010 Apr;19(4):631-41. doi: 10.1002/pro.344. Protein Sci. 2010. PMID: 20095051 Free PMC article. Review.
Cited by
-
What makes proteins work: exploring life in P-T-X.Phys Biol. 2016 Nov 15;13(6):063001. doi: 10.1088/1478-3975/13/6/063001. Phys Biol. 2016. PMID: 27845917 Free PMC article.
-
Cavity as a source of conformational fluctuation and high-energy state: high-pressure NMR study of a cavity-enlarged mutant of T4 lysozyme.Biophys J. 2015 Jan 6;108(1):133-45. doi: 10.1016/j.bpj.2014.11.012. Biophys J. 2015. PMID: 25564860 Free PMC article.
-
Protein unfolded states populated at high and ambient pressure are similarly compact.Biophys J. 2021 Jun 15;120(12):2592-2598. doi: 10.1016/j.bpj.2021.04.031. Epub 2021 May 4. Biophys J. 2021. PMID: 33961866 Free PMC article.
-
Single-crystal CVD diamonds as small-angle X-ray scattering windows for high-pressure research.J Appl Crystallogr. 2012 Jun 1;45(Pt 3):453-457. doi: 10.1107/S0021889812010722. Epub 2012 Apr 25. J Appl Crystallogr. 2012. PMID: 22675230 Free PMC article.
-
Lessons from pressure denaturation of proteins.J R Soc Interface. 2018 Oct 3;15(147):20180244. doi: 10.1098/rsif.2018.0244. J R Soc Interface. 2018. PMID: 30282759 Free PMC article. Review.
References
-
- Collins MD, Hummer G, Quillin ML, Matthews BW, Gruner SM. Cooperative water filling of a nonpolar protein cavity observed by high-pressure crystallography and simulation. Proc. Nat. Acad. Sci U.S.A. 2005;102:16668–16671. [published erratum appears in (2006) Proc. Nat. Acad. Sci U.S.A. 103, 4793] - PMC - PubMed
-
- Dill KA, Shortle D. Denatured states of proteins. Annu. Rev. Biochem. 1991;60:795–825. - PubMed
-
- Kauzmann W. Denaturation of proteins and enzymes. In: McElroy WD, Glass B, editors. The mechanism of enzyme action. Johns Hopkins Press; Baltimore, MD: 1954. pp. 70–110.
-
- Kauzmann W. Some factors in the interpretation of protein denaturation. Adv. Protein Chem. 1959;14:1–63. - PubMed
-
- Dill KA. Dominant forces in protein folding. Biochemistry. 1990;29:7133–7155. - PubMed
Publication types
MeSH terms
Substances
Grants and funding
LinkOut - more resources
Full Text Sources
Research Materials
Miscellaneous