Regulation of dynactin through the differential expression of p150Glued isoforms
- PMID: 18812314
- PMCID: PMC2586252
- DOI: 10.1074/jbc.M804840200
Regulation of dynactin through the differential expression of p150Glued isoforms
Abstract
Cytoplasmic dynein and dynactin interact to drive microtubule-based transport in the cell. The p150Glued subunit of dynactin binds to dynein, and directly to microtubules. We have identified alternatively spliced isoforms of p150Glued that are expressed in a tissue-specific manner and which differ significantly in their affinity for microtubules. Live cell assays indicate that these alternatively spliced isoforms also differ significantly in their microtubule plus end-tracking activity, suggesting a mechanism by which the cell may regulate the dynamic localization of dynactin. To test the function of the microtubule-binding domain of p150Glued, we used RNAi to deplete the endogenous polypeptide from HeLa cells, followed by rescue with constructs encoding either the full-length polypeptide or an isoform lacking the microtubule-binding domain. Both constructs fully rescued defects in Golgi morphology induced by depletion of p150Glued, indicating that an independent microtubule-binding site in dynactin may not be required for dynactin-mediated trafficking in some mammalian cell types. In neurons, however, a mutation within the microtubule-binding domain of p150Glued results in motor neuron disease; here we investigate the effects of four other mutations in highly conserved domains of the polypeptide (M571T, R785W, R1101K, and T1249I) associated in genetic studies with Amyotrophic Lateral Sclerosis. Both biochemical and cellular assays reveal that these amino acid substitutions do not result in functional differences, suggesting that these sequence changes are either allelic variants or contributory risk factors rather than causative for motor neuron disease. Together, these studies provide further insight into the regulation of dynein-dynactin function in the cell.
Figures
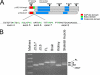
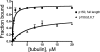
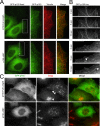
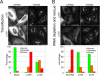
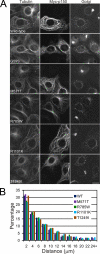
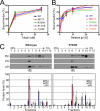
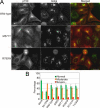
Similar articles
-
The p150Glued component of the dynactin complex binds to both microtubules and the actin-related protein centractin (Arp-1).Proc Natl Acad Sci U S A. 1995 Feb 28;92(5):1634-8. doi: 10.1073/pnas.92.5.1634. Proc Natl Acad Sci U S A. 1995. PMID: 7878030 Free PMC article.
-
Dynactin subunit p150Glued isoforms notable for differential interaction with microtubules.Traffic. 2009 Nov;10(11):1635-46. doi: 10.1111/j.1600-0854.2009.00976.x. Epub 2009 Aug 10. Traffic. 2009. PMID: 19778315
-
A motor neuron disease-associated mutation in p150Glued perturbs dynactin function and induces protein aggregation.J Cell Biol. 2006 Feb 27;172(5):733-45. doi: 10.1083/jcb.200511068. J Cell Biol. 2006. PMID: 16505168 Free PMC article.
-
The role of the dynactin complex in intracellular motility.Int Rev Cytol. 1998;182:69-109. doi: 10.1016/s0074-7696(08)62168-3. Int Rev Cytol. 1998. PMID: 9522459 Review.
-
[Dynein and dynactin as organizers of the system of cell microtubules].Ontogenez. 2006 Sep-Oct;37(5):323-39. Ontogenez. 2006. PMID: 17066975 Review. Russian.
Cited by
-
Dynactin is required for transport initiation from the distal axon.Neuron. 2012 Apr 26;74(2):331-43. doi: 10.1016/j.neuron.2012.02.025. Neuron. 2012. PMID: 22542186 Free PMC article.
-
Perry Disease: Concept of a New Disease and Clinical Diagnostic Criteria.J Mov Disord. 2021 Jan;14(1):1-9. doi: 10.14802/jmd.20060. Epub 2020 Sep 21. J Mov Disord. 2021. PMID: 32942840 Free PMC article.
-
BPAG1a and b associate with EB1 and EB3 and modulate vesicular transport, Golgi apparatus structure, and cell migration in C2.7 myoblasts.PLoS One. 2014 Sep 22;9(9):e107535. doi: 10.1371/journal.pone.0107535. eCollection 2014. PLoS One. 2014. PMID: 25244344 Free PMC article.
-
Evolution of the eukaryotic dynactin complex, the activator of cytoplasmic dynein.BMC Evol Biol. 2012 Jun 22;12:95. doi: 10.1186/1471-2148-12-95. BMC Evol Biol. 2012. PMID: 22726940 Free PMC article.
-
Dynactin subunit p150(Glued) is a neuron-specific anti-catastrophe factor.PLoS Biol. 2013 Jul;11(7):e1001611. doi: 10.1371/journal.pbio.1001611. Epub 2013 Jul 16. PLoS Biol. 2013. PMID: 23874158 Free PMC article.
References
-
- Hafezparast, M., Klocke, R., Ruhrberg, C., Marquardt, A., Ahmad-Annuar, A., Bowen, S., Lalli, G., Witherden, A. S., Hummerich, H., Nicholson, S., Morgan, P. J., Oozageer, R., Priestley, J. V., Averill, S., King, V. R., Ball, S., Peters, J., Toda, T., Yamamoto, A., Hiraoka, Y., Augustin, M., Korthaus, D., Wattler, S., Wabnitz, P., Dickneite, C., Lampel, S., Boehme, F., Peraus, G., Popp, A., Rudelius, M., Schlegel, J., Fuchs, H., Hrabe de Angelis, M., Schiavo, G., Shima, D. T., Russ, A. P., Stumm, G., Martin, J. E., and Fisher, E. M. (2003) Science 300 808-812 - PubMed
-
- Puls, I., Jonnakuty, C., LaMonte, B. H., Holzbaur, E. L., Tokito, M., Mann, E., Floeter, M. K., Bidus, K., Drayna, D., Oh, S. J., Brown, R. H., Jr., Ludlow, C. L., and Fischbeck, K. H. (2003) Nat. Genet. 33 455-456 - PubMed
Publication types
MeSH terms
Substances
Grants and funding
LinkOut - more resources
Full Text Sources