TSC-mTOR maintains quiescence and function of hematopoietic stem cells by repressing mitochondrial biogenesis and reactive oxygen species
- PMID: 18809716
- PMCID: PMC2556783
- DOI: 10.1084/jem.20081297
TSC-mTOR maintains quiescence and function of hematopoietic stem cells by repressing mitochondrial biogenesis and reactive oxygen species
Abstract
The tuberous sclerosis complex (TSC)-mammalian target of rapamycin (mTOR) pathway is a key regulator of cellular metabolism. We used conditional deletion of Tsc1 to address how quiescence is associated with the function of hematopoietic stem cells (HSCs). We demonstrate that Tsc1 deletion in the HSCs drives them from quiescence into rapid cycling, with increased mitochondrial biogenesis and elevated levels of reactive oxygen species (ROS). Importantly, this deletion dramatically reduced both hematopoiesis and self-renewal of HSCs, as revealed by serial and competitive bone marrow transplantation. In vivo treatment with an ROS antagonist restored HSC numbers and functions. These data demonstrated that the TSC-mTOR pathway maintains the quiescence and function of HSCs by repressing ROS production. The detrimental effect of up-regulated ROS in metabolically active HSCs may explain the well-documented association between quiescence and the "stemness" of HSCs.
Figures
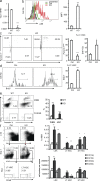
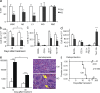
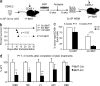
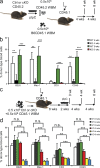
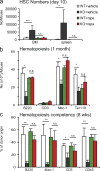
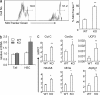
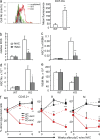
Similar articles
-
The axis of mTOR-mitochondria-ROS and stemness of the hematopoietic stem cells.Cell Cycle. 2009 Apr 15;8(8):1158-60. doi: 10.4161/cc.8.8.8139. Epub 2009 Apr 10. Cell Cycle. 2009. PMID: 19270502
-
A low level of reactive oxygen species selects for primitive hematopoietic stem cells that may reside in the low-oxygenic niche.Blood. 2007 Oct 15;110(8):3056-63. doi: 10.1182/blood-2007-05-087759. Epub 2007 Jun 26. Blood. 2007. PMID: 17595331 Free PMC article.
-
A complex interplay between PGC-1 co-activators and mTORC1 regulates hematopoietic recovery following 5-fluorouracil treatment.Stem Cell Res. 2014 Jan;12(1):178-93. doi: 10.1016/j.scr.2013.10.006. Epub 2013 Oct 24. Stem Cell Res. 2014. PMID: 24239965
-
Cited2 in hematopoietic stem cell function.Curr Opin Hematol. 2013 Jul;20(4):301-7. doi: 10.1097/MOH.0b013e3283606022. Curr Opin Hematol. 2013. PMID: 23507959 Review.
-
Role of the mammalian target of rapamycin pathway in lentiviral vector transduction of hematopoietic stem cells.Curr Opin Hematol. 2015 Jul;22(4):302-8. doi: 10.1097/MOH.0000000000000150. Curr Opin Hematol. 2015. PMID: 26049750 Free PMC article. Review.
Cited by
-
Biting the hand that feeds: Metabolic determinants of cell fate during infection.Front Immunol. 2022 Oct 13;13:923024. doi: 10.3389/fimmu.2022.923024. eCollection 2022. Front Immunol. 2022. PMID: 36311735 Free PMC article. Review.
-
Akt-signal integration is involved in the differentiation of embryonal carcinoma cells.PLoS One. 2013 Jun 6;8(6):e64877. doi: 10.1371/journal.pone.0064877. Print 2013. PLoS One. 2013. PMID: 23762260 Free PMC article.
-
Metabolic Regulation of Thymic Epithelial Cell Function.Front Immunol. 2021 Mar 3;12:636072. doi: 10.3389/fimmu.2021.636072. eCollection 2021. Front Immunol. 2021. PMID: 33746975 Free PMC article. Review.
-
Metabolism in Hematopoiesis and Its Malignancy.Adv Exp Med Biol. 2023;1442:45-64. doi: 10.1007/978-981-99-7471-9_4. Adv Exp Med Biol. 2023. PMID: 38228958
-
Gene regulatory networks controlling hematopoietic progenitor niche cell production and differentiation in the Drosophila lymph gland.PLoS One. 2012;7(7):e41604. doi: 10.1371/journal.pone.0041604. Epub 2012 Jul 24. PLoS One. 2012. PMID: 22911822 Free PMC article.
References
-
- Ogawa, M. 1993. Differentiation and proliferation of hematopoietic stem cells. Blood. 81:2844–2853. - PubMed
-
- Lajtha, L.G. 1963. On the concept of the cell cycle. J. Cell. Physiol. 62(Suppl. 1):143–145. - PubMed
-
- Lajtha, L.G. 1979. Stem cell concepts. Differentiation. 14:23–34. - PubMed
-
- Zhang, J., C. Niu, L. Ye, H. Huang, X. He, W.G. Tong, J. Ross, J. Haug, T. Johnson, J.Q. Feng, et al. 2003. Identification of the haematopoietic stem cell niche and control of the niche size. Nature. 425:836–841. - PubMed
-
- Cheng, T., N. Rodrigues, H. Shen, Y. Yang, D. Dombkowski, M. Sykes, and D.T. Scadden. 2000. Hematopoietic stem cell quiescence maintained by p21cip1/waf1. Science. 287:1804–1808. - PubMed
Publication types
MeSH terms
Substances
LinkOut - more resources
Full Text Sources
Other Literature Sources
Medical
Molecular Biology Databases
Miscellaneous