Inhibition of dendritic cell differentiation and accumulation of myeloid-derived suppressor cells in cancer is regulated by S100A9 protein
- PMID: 18809714
- PMCID: PMC2556797
- DOI: 10.1084/jem.20080132
Inhibition of dendritic cell differentiation and accumulation of myeloid-derived suppressor cells in cancer is regulated by S100A9 protein
Abstract
Accumulation of myeloid-derived suppressor cells (MDSCs) associated with inhibition of dendritic cell (DC) differentiation is one of the major immunological abnormalities in cancer and leads to suppression of antitumor immune responses. The molecular mechanism of this phenomenon remains unclear. We report here that STAT3-inducible up-regulation of the myeloid-related protein S100A9 enhances MDSC production in cancer. Mice lacking this protein mounted potent antitumor immune responses and rejected implanted tumors. This effect was reversed by administration of wild-type MDSCs from tumor-bearing mice to S100A9-null mice. Overexpression of S100A9 in cultured embryonic stem cells or transgenic mice inhibited the differentiation of DCs and macrophages and induced accumulation of MDSCs. This study demonstrates that tumor-induced up-regulation of S100A9 protein is critically important for accumulation of MDSCs and reveals a novel molecular mechanism of immunological abnormalities in cancer.
Figures
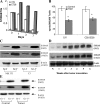
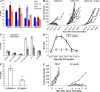
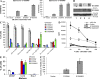
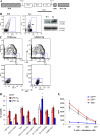
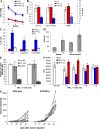
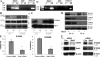
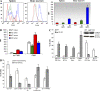
Similar articles
-
Liver X receptor regulates mouse GM-CSF-derived dendritic cell differentiation in vitro.Mol Immunol. 2014 Jul;60(1):32-43. doi: 10.1016/j.molimm.2014.03.006. Epub 2014 Apr 18. Mol Immunol. 2014. PMID: 24747959
-
Up-regulated myeloid-derived suppressor cell contributes to hepatocellular carcinoma development by impairing dendritic cell function.Scand J Gastroenterol. 2011 Feb;46(2):156-64. doi: 10.3109/00365521.2010.516450. Epub 2010 Sep 7. Scand J Gastroenterol. 2011. PMID: 20822377
-
IRF7 regulates the development of granulocytic myeloid-derived suppressor cells through S100A9 transrepression in cancer.Oncogene. 2017 May 25;36(21):2969-2980. doi: 10.1038/onc.2016.448. Epub 2017 Jan 16. Oncogene. 2017. PMID: 28092673
-
Signaling pathways involved in MDSC regulation.Biochim Biophys Acta. 2014 Aug;1846(1):55-65. doi: 10.1016/j.bbcan.2014.04.003. Epub 2014 Apr 13. Biochim Biophys Acta. 2014. PMID: 24727385 Free PMC article. Review.
-
STAT3 in Tumor-Associated Myeloid Cells: Multitasking to Disrupt Immunity.Int J Mol Sci. 2018 Jun 19;19(6):1803. doi: 10.3390/ijms19061803. Int J Mol Sci. 2018. PMID: 29921770 Free PMC article. Review.
Cited by
-
The pre-metastatic niche: is metastasis random?Bonekey Rep. 2012 May 2;1:80. doi: 10.1038/bonekey.2012.80. eCollection 2012. Bonekey Rep. 2012. PMID: 27127624 Free PMC article. Review.
-
Chemoimmunomodulation of MDSCs as a novel strategy for cancer therapy.Oncoimmunology. 2012 Jan 1;1(1):121-122. doi: 10.4161/onci.1.1.18074. Oncoimmunology. 2012. PMID: 22720231 Free PMC article.
-
Exploiting innate immunity for cancer immunotherapy.Mol Cancer. 2023 Nov 27;22(1):187. doi: 10.1186/s12943-023-01885-w. Mol Cancer. 2023. PMID: 38008741 Free PMC article. Review.
-
Anti-GD2 mAb and Vorinostat synergize in the treatment of neuroblastoma.Oncoimmunology. 2016 Mar 28;5(6):e1164919. doi: 10.1080/2162402X.2016.1164919. eCollection 2016 Jun. Oncoimmunology. 2016. PMID: 27471639 Free PMC article.
-
Deregulation of apoptotic factors Bcl-xL and Bax confers apoptotic resistance to myeloid-derived suppressor cells and contributes to their persistence in cancer.J Biol Chem. 2013 Jun 28;288(26):19103-15. doi: 10.1074/jbc.M112.434530. Epub 2013 May 15. J Biol Chem. 2013. PMID: 23677993 Free PMC article.
References
-
- Gabrilovich, D.I. 2004. The mechanisms and functional significance of tumour-induced dendritic-cell defects. Nat. Rev. Immunol. 4:941–952. - PubMed
-
- Serafini, P., I. Borrello, and V. Bronte. 2006. Myeloid suppressor cells in cancer: recruitment, phenotype, properties, and mechanisms of immune suppression. Semin. Cancer Biol. 16:53–65. - PubMed
-
- Ochoa, A.C., A.H. Zea, C. Hernandez, and P.C. Rodriguez. 2007. Arginase, prostaglandins, and myeloid-derived suppressor cells in renal cell carcinoma. Clin. Cancer Res. 13:721s–726s. - PubMed
Publication types
MeSH terms
Substances
Grants and funding
LinkOut - more resources
Full Text Sources
Other Literature Sources
Molecular Biology Databases
Research Materials
Miscellaneous