UCP2 modulates cell proliferation through the MAPK/ERK pathway during erythropoiesis and has no effect on heme biosynthesis
- PMID: 18687678
- PMCID: PMC2576537
- DOI: 10.1074/jbc.M805400200
UCP2 modulates cell proliferation through the MAPK/ERK pathway during erythropoiesis and has no effect on heme biosynthesis
Abstract
UCP2, an inner membrane mitochondrial protein, has been implicated in bioenergetics and reactive oxygen species (ROS) modulation. High levels of UCP2 mRNA were recently found in erythroid cells where UCP2 is hypothesized to function as a facilitator of heme synthesis and iron metabolism by reducing ROS production. We examined UCP2 protein expression and role in mice erythropoiesis in vivo. UCP2 was mainly expressed at early stages of erythroid maturation when cells are not fully committed in heme synthesis. Iron incorporation into heme was unaltered in reticulocytes from UCP2-deficient mice. Although heme synthesis was not influenced by UCP2 deficiency, mice lacking UCP2 had a delayed recovery from chemically induced hemolytic anemia. Analysis of progenitor cells from bone marrow and fetal liver both in vitro and in vivo revealed that UCP2 deficiency results in a significant decrease in cell proliferation at the erythropoietin-dependent phase of erythropoiesis. This was accompanied by reduction in the phosphorylated form of ERK, a ROS-dependent cytosolic regulator of cell proliferation. Analysis of ROS in UCP2 null erythroid cells revealed altered distribution of ROS, resulting in decreased cytosolic and increased mitochondrial ROS. Restoration of the cytosol oxidative state of erythroid progenitor cells by the pro-oxidant Paraquat reversed the effect of UCP2 deficiency on cell proliferation in in vitro differentiation assays. Together, these results indicate that UCP2 is a regulator of erythropoiesis and suggests that inhibition of UCP2 function may contribute to the development of anemia.
Figures
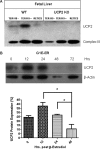
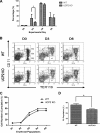
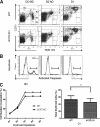
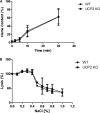
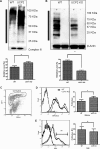
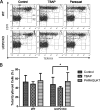
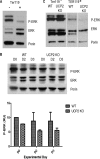
Similar articles
-
FAM210B is an erythropoietin target and regulates erythroid heme synthesis by controlling mitochondrial iron import and ferrochelatase activity.J Biol Chem. 2018 Dec 21;293(51):19797-19811. doi: 10.1074/jbc.RA118.002742. Epub 2018 Oct 26. J Biol Chem. 2018. PMID: 30366982 Free PMC article.
-
Stat3 mediates LIF-induced protection of astrocytes against toxic ROS by upregulating the UPC2 mRNA pool.Glia. 2014 Feb;62(2):159-70. doi: 10.1002/glia.22594. Epub 2013 Dec 5. Glia. 2014. PMID: 24307565
-
Increased activity of mitochondrial uncoupling protein 2 improves stress resistance in cultured endothelial cells exposed in vitro to high glucose levels.Am J Physiol Heart Circ Physiol. 2015 Jul 1;309(1):H147-56. doi: 10.1152/ajpheart.00759.2014. Epub 2015 Apr 24. Am J Physiol Heart Circ Physiol. 2015. PMID: 25910810
-
Regulation of erythroid 5-aminolevulinate synthase expression during erythropoiesis.Int J Biochem Cell Biol. 1999 Oct;31(10):1153-67. doi: 10.1016/s1357-2725(99)00073-4. Int J Biochem Cell Biol. 1999. PMID: 10582344 Review.
-
Ineffective erythropoiesis and thalassemias.Curr Opin Hematol. 2009 May;16(3):187-94. doi: 10.1097/MOH.0b013e32832990a4. Curr Opin Hematol. 2009. PMID: 19318943 Free PMC article. Review.
Cited by
-
Molecular Pathways Involved in the Development of Congenital Erythrocytosis.Genes (Basel). 2021 Jul 28;12(8):1150. doi: 10.3390/genes12081150. Genes (Basel). 2021. PMID: 34440324 Free PMC article. Review.
-
Cerium Oxide Nanoparticle Administration to Skeletal Muscle Cells under Different Gravity and Radiation Conditions.ACS Appl Mater Interfaces. 2021 Sep 1;13(34):40200-40213. doi: 10.1021/acsami.1c14176. Epub 2021 Aug 19. ACS Appl Mater Interfaces. 2021. PMID: 34410709 Free PMC article.
-
ErbB1/2 tyrosine kinase inhibitor mediates oxidative stress-induced apoptosis in inflammatory breast cancer cells.Breast Cancer Res Treat. 2012 Feb;132(1):109-19. doi: 10.1007/s10549-011-1568-1. Epub 2011 May 11. Breast Cancer Res Treat. 2012. PMID: 21559822 Free PMC article.
-
Mitochondrial Uncoupling Proteins: Subtle Regulators of Cellular Redox Signaling.Antioxid Redox Signal. 2018 Sep 1;29(7):667-714. doi: 10.1089/ars.2017.7225. Epub 2018 Mar 14. Antioxid Redox Signal. 2018. PMID: 29351723 Free PMC article. Review.
-
Quercetin Affects Erythropoiesis and Heart Mitochondrial Function in Mice.Oxid Med Cell Longev. 2015;2015:836301. doi: 10.1155/2015/836301. Epub 2015 May 28. Oxid Med Cell Longev. 2015. PMID: 26106459 Free PMC article.
References
-
- Sluse, F. E., Jarmuszkiewicz, W., Navet, R., Douette, P., Mathy, G., and Sluse-Goffart, C. M. (2006) Biochim. Biophys. Acta 1757 480–485 - PubMed
-
- Esteves, T. C., and Brand, M. D. (2005) Biochim. Biophys. Acta 1709 35–44 - PubMed
-
- Ricquier, D., Miroux, B., Larose, M., Cassard-Doulcier, A. M., and Bouillaud, F. (2000) Int. J. Obes. Relat. Metab. Disord. 24 Suppl. 2, 86–88 - PubMed
-
- Nicholls, D. G. (2006) Biochim. Biophys. Acta 1757 459–466 - PubMed
-
- Krauss, S., Zhang, C. Y., and Lowell, B. B. (2005) Nat. Rev. Mol. Cell Biol. 6 248–261 - PubMed
Publication types
MeSH terms
Substances
Grants and funding
LinkOut - more resources
Full Text Sources
Medical
Molecular Biology Databases
Miscellaneous