Raf activation is regulated by tyrosine 510 phosphorylation in Drosophila
- PMID: 18494562
- PMCID: PMC2386837
- DOI: 10.1371/journal.pbio.0060128
Raf activation is regulated by tyrosine 510 phosphorylation in Drosophila
Abstract
The proto-oncoprotein Raf is pivotal for mitogen-activated protein kinase (MAPK) signaling, and its aberrant activation has been implicated in multiple human cancers. However, the precise molecular mechanism of Raf activation, especially for B-Raf, remains unresolved. By genetic and biochemical studies, we demonstrate that phosphorylation of tyrosine 510 is essential for activation of Drosophila Raf (Draf), which is an ortholog of mammalian B-Raf. Y510 of Draf is phosphorylated by the c-src homolog Src64B. Acidic substitution of Y510 promotes and phenylalanine substitution impairs Draf activation without affecting its enzymatic activity, suggesting that Y510 plays a purely regulatory role. We further show that Y510 regulates Draf activation by affecting the autoinhibitory interaction between the N- and C-terminal fragments of the protein. Finally, we show that Src64B is required for Draf activation in several developmental processes. Together, these results suggest a novel mechanism of Raf activation via Src-mediated tyrosine phosphorylation. Since Y510 is a conserved residue in the kinase domain of all Raf proteins, this mechanism is likely evolutionarily conserved.
Conflict of interest statement
Figures
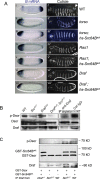
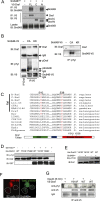
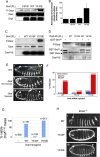
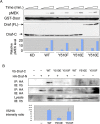
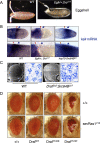
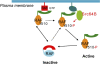
Similar articles
-
A novel conserved phosphotyrosine motif in the Drosophila fibroblast growth factor signaling adaptor Dof with a redundant role in signal transmission.Mol Cell Biol. 2010 Apr;30(8):2017-27. doi: 10.1128/MCB.01436-09. Epub 2010 Feb 12. Mol Cell Biol. 2010. PMID: 20154139 Free PMC article.
-
Src42 binding activity regulates Drosophila RAF by a novel CNK-dependent derepression mechanism.EMBO J. 2005 Feb 9;24(3):487-98. doi: 10.1038/sj.emboj.7600558. Epub 2005 Jan 20. EMBO J. 2005. PMID: 15660123 Free PMC article.
-
Identification of autosomal regions involved in Drosophila Raf function.Genetics. 2000 Oct;156(2):763-74. doi: 10.1093/genetics/156.2.763. Genetics. 2000. PMID: 11014822 Free PMC article.
-
Ras activation of the Raf kinase: tyrosine kinase recruitment of the MAP kinase cascade.Recent Prog Horm Res. 2001;56:127-55. doi: 10.1210/rp.56.1.127. Recent Prog Horm Res. 2001. PMID: 11237210 Review.
-
Mechanisms regulating Raf-1 activity in signal transduction pathways.Mol Reprod Dev. 1995 Dec;42(4):507-14. doi: 10.1002/mrd.1080420420. Mol Reprod Dev. 1995. PMID: 8607983 Review.
Cited by
-
Functions and Mechanisms of Fibroblast Growth Factor (FGF) Signalling in Drosophila melanogaster.Int J Mol Sci. 2013 Mar 14;14(3):5920-37. doi: 10.3390/ijms14035920. Int J Mol Sci. 2013. PMID: 23493057 Free PMC article.
-
Synthetic approaches to protein phosphorylation.Curr Opin Chem Biol. 2015 Oct;28:115-22. doi: 10.1016/j.cbpa.2015.07.001. Epub 2015 Jul 18. Curr Opin Chem Biol. 2015. PMID: 26196731 Free PMC article. Review.
-
A novel conserved phosphotyrosine motif in the Drosophila fibroblast growth factor signaling adaptor Dof with a redundant role in signal transmission.Mol Cell Biol. 2010 Apr;30(8):2017-27. doi: 10.1128/MCB.01436-09. Epub 2010 Feb 12. Mol Cell Biol. 2010. PMID: 20154139 Free PMC article.
-
Co-dependent regulation of p-BRAF and potassium channel KCNMA1 levels drives glioma progression.Cell Mol Life Sci. 2023 Feb 10;80(3):61. doi: 10.1007/s00018-023-04708-9. Cell Mol Life Sci. 2023. PMID: 36763212 Free PMC article.
-
The chemical biology of protein phosphorylation.Annu Rev Biochem. 2009;78:797-825. doi: 10.1146/annurev.biochem.78.070907.103047. Annu Rev Biochem. 2009. PMID: 19489734 Free PMC article. Review.
References
-
- Widmann C, Gibson S, Jarpe MB, Johnson GL. Mitogen-activated protein kinase: conservation of a three-kinase module from yeast to human. Physiol Rev. 1999;79:143–180. - PubMed
-
- Seger R, Krebs EG. The MAPK signaling cascade. FASEB J. 1995;9:726–735. - PubMed
-
- Davies H, Bignell GR, Cox C, Stephens P, Edkins S, et al. Mutations of the BRAF gene in human cancer. Nature. 2002;417:949–954. - PubMed
-
- Rajagopalan H, Bardelli A, Lengauer C, Kinzler KW, Vogelstein B, et al. Tumorigenesis: RAF/RAS oncogenes and mismatch-repair status. Nature. 2002;418:934. - PubMed
-
- Morrison DK, Cutler RE. The complexity of Raf-1 regulation. Curr Opin Cell Biol. 1997;9:174–179. - PubMed
Publication types
MeSH terms
Substances
Grants and funding
LinkOut - more resources
Full Text Sources
Molecular Biology Databases
Research Materials
Miscellaneous