Vision triggers an experience-dependent sensitive period at the retinogeniculate synapse
- PMID: 18448657
- PMCID: PMC2793334
- DOI: 10.1523/JNEUROSCI.4667-07.2008
Vision triggers an experience-dependent sensitive period at the retinogeniculate synapse
Abstract
In the mammalian visual system, sensory experience is widely thought to sculpt cortical circuits during a precise critical period. In contrast, subcortical regions, such as the thalamus, were thought to develop at earlier ages in a vision-independent manner. Recent studies at the retinogeniculate synapse, however, have demonstrated an influence of vision on the formation of synaptic circuits in the thalamus. In mice, dark rearing from birth does not alter normal developmental maturation of the connection between retina and thalamus. However, deprivation 20 d after birth [postnatal day 20 (p20)] resulted in dramatic weakening of synaptic strength and an increase in the number of retinal inputs that innervate a thalamic relay neuron. Here, by quantifying changes in synaptic strength and connectivity in response to different time windows of deprivation, we find that several days of vision after eye opening is necessary for triggering experience-dependent plasticity. Shorter periods of visual experience do not permit similar experience-dependent synaptic reorganization. Furthermore, changes in connectivity are rapidly reversible simply by restoring normal vision. However, similar plasticity did not occur when shifting the onset of deprivation to p25. Although synapses still weakened, recruitment of additional retinal inputs no longer occurred. Therefore, synaptic circuits in the visual thalamus are unexpectedly malleable during a late developmental period, after the time when normal synapse elimination and pruning has occurred. This thalamic sensitive period overlaps temporally with experience-dependent changes in the cortex, suggesting that subcortical plasticity may influence cortical responses to sensory experience.
Figures
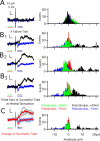
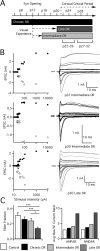
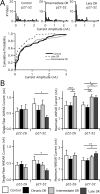
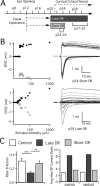
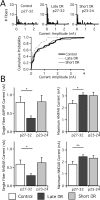
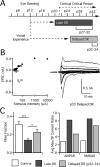
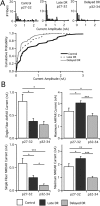
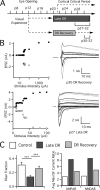
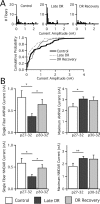
Similar articles
-
Different roles for AMPA and NMDA receptors in transmission at the immature retinogeniculate synapse.J Neurophysiol. 2008 Feb;99(2):629-43. doi: 10.1152/jn.01171.2007. Epub 2007 Nov 21. J Neurophysiol. 2008. PMID: 18032559
-
Changes in input strength and number are driven by distinct mechanisms at the retinogeniculate synapse.J Neurophysiol. 2014 Aug 15;112(4):942-50. doi: 10.1152/jn.00175.2014. Epub 2014 May 21. J Neurophysiol. 2014. PMID: 24848465 Free PMC article.
-
Distinct roles for spontaneous and visual activity in remodeling of the retinogeniculate synapse.Neuron. 2006 Oct 19;52(2):281-91. doi: 10.1016/j.neuron.2006.07.007. Neuron. 2006. PMID: 17046691
-
Circuitry Underlying Experience-Dependent Plasticity in the Mouse Visual System.Neuron. 2020 Apr 8;106(1):21-36. doi: 10.1016/j.neuron.2020.01.031. Neuron. 2020. PMID: 32272065 Free PMC article. Review.
-
An evolving view of retinogeniculate transmission.Vis Neurosci. 2017 Jan;34:E013. doi: 10.1017/S0952523817000104. Vis Neurosci. 2017. PMID: 28965513 Free PMC article. Review.
Cited by
-
Loss of Retinogeniculate Synaptic Function in the DBA/2J Mouse Model of Glaucoma.eNeuro. 2022 Dec 27;9(6):ENEURO.0421-22.2022. doi: 10.1523/ENEURO.0421-22.2022. Print 2022 Nov-Dec. eNeuro. 2022. PMID: 36526366 Free PMC article.
-
Structural and Functional Plasticity in the Dorsolateral Geniculate Nucleus of Mice following Bilateral Enucleation.Neuroscience. 2022 Apr 15;488:44-59. doi: 10.1016/j.neuroscience.2022.01.029. Epub 2022 Feb 4. Neuroscience. 2022. PMID: 35131394 Free PMC article.
-
Identifying roles for neurotransmission in circuit assembly: insights gained from multiple model systems and experimental approaches.Bioessays. 2011 Jan;33(1):61-72. doi: 10.1002/bies.201000095. Bioessays. 2011. PMID: 21110347 Free PMC article. Review.
-
An activity-dependent determinant of synapse elimination in the mammalian brain.Neuron. 2021 Apr 21;109(8):1333-1349.e6. doi: 10.1016/j.neuron.2021.03.006. Epub 2021 Mar 25. Neuron. 2021. PMID: 33770504 Free PMC article.
-
Visual deprivation increases accumulation of dense core vesicles in developing optic tectal synapses in Xenopus laevis.J Comp Neurol. 2010 Jun 15;518(12):2365-81. doi: 10.1002/cne.22338. J Comp Neurol. 2010. PMID: 20437533 Free PMC article.
References
-
- Akerman CJ, Smyth D, Thompson ID. Visual experience before eye-opening and the development of the retinogeniculate pathway. Neuron. 2002;36:869–879. - PubMed
-
- Alexander GM, Fisher TL, Godwin DW. Differential response dynamics of corticothalamic glutamatergic synapses in the lateral geniculate nucleus and thalamic reticular nucleus. Neuroscience. 2006;137:367–372. - PubMed
-
- Antonini A, Stryker MP. Rapid remodeling of axonal arbors in the visual cortex. Science. 1993;260:1819–1821. - PubMed
-
- Burrone J, O'Byrne M, Murthy VN. Multiple forms of synaptic plasticity triggered by selective suppression of activity in individual neurons. Nature. 2002;420:414–418. - PubMed
Publication types
MeSH terms
Grants and funding
- F31 NS048630/NS/NINDS NIH HHS/United States
- R01 EY013613-03/EY/NEI NIH HHS/United States
- R01 EY013613-04/EY/NEI NIH HHS/United States
- F31 NS048630-03/NS/NINDS NIH HHS/United States
- R01 EY013613-05/EY/NEI NIH HHS/United States
- F31 NS048630-02/NS/NINDS NIH HHS/United States
- R01EY013613/EY/NEI NIH HHS/United States
- P30 HD018655-26/HD/NICHD NIH HHS/United States
- P01HD18655/HD/NICHD NIH HHS/United States
- R01 EY013613/EY/NEI NIH HHS/United States
- P30 HD018655-25/HD/NICHD NIH HHS/United States
- P30 HD018655/HD/NICHD NIH HHS/United States
- F31 NS048630-01/NS/NINDS NIH HHS/United States
LinkOut - more resources
Full Text Sources