Structural analysis of major species barriers between humans and palm civets for severe acute respiratory syndrome coronavirus infections
- PMID: 18448527
- PMCID: PMC2446986
- DOI: 10.1128/JVI.00442-08
Structural analysis of major species barriers between humans and palm civets for severe acute respiratory syndrome coronavirus infections
Abstract
It is believed that a novel coronavirus, severe acute respiratory syndrome coronavirus (SARS-CoV), was passed from palm civets to humans and caused the epidemic of SARS in 2002 to 2003. The major species barriers between humans and civets for SARS-CoV infections are the specific interactions between a defined receptor-binding domain (RBD) on a viral spike protein and its host receptor, angiotensin-converting enzyme 2 (ACE2). In this study a chimeric ACE2 bearing the critical N-terminal helix from civet and the remaining peptidase domain from human was constructed, and it was shown that this construct has the same receptor activity as civet ACE2. In addition, crystal structures of the chimeric ACE2 complexed with RBDs from various human and civet SARS-CoV strains were determined. These structures, combined with a previously determined structure of human ACE2 complexed with the RBD from a human SARS-CoV strain, have revealed a structural basis for understanding the major species barriers between humans and civets for SARS-CoV infections. They show that the major species barriers are determined by interactions between four ACE2 residues (residues 31, 35, 38, and 353) and two RBD residues (residues 479 and 487), that early civet SARS-CoV isolates were prevented from infecting human cells due to imbalanced salt bridges at the hydrophobic virus/receptor interface, and that SARS-CoV has evolved to gain sustained infectivity for human cells by eliminating unfavorable free charges at the interface through stepwise mutations at positions 479 and 487. These results enhance our understanding of host adaptations and cross-species infections of SARS-CoV and other emerging animal viruses.
Figures
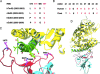
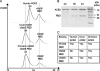
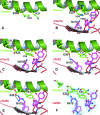
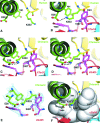
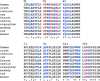
Similar articles
-
Mechanisms of host receptor adaptation by severe acute respiratory syndrome coronavirus.J Biol Chem. 2012 Mar 16;287(12):8904-11. doi: 10.1074/jbc.M111.325803. Epub 2012 Jan 30. J Biol Chem. 2012. PMID: 22291007 Free PMC article.
-
Pathways of cross-species transmission of synthetically reconstructed zoonotic severe acute respiratory syndrome coronavirus.J Virol. 2008 Sep;82(17):8721-32. doi: 10.1128/JVI.00818-08. Epub 2008 Jun 25. J Virol. 2008. PMID: 18579604 Free PMC article.
-
Structure of SARS coronavirus spike receptor-binding domain complexed with receptor.Science. 2005 Sep 16;309(5742):1864-8. doi: 10.1126/science.1116480. Science. 2005. PMID: 16166518
-
Receptor recognition and cross-species infections of SARS coronavirus.Antiviral Res. 2013 Oct;100(1):246-54. doi: 10.1016/j.antiviral.2013.08.014. Epub 2013 Aug 29. Antiviral Res. 2013. PMID: 23994189 Free PMC article. Review.
-
Insights from the association of SARS-CoV S-protein with its receptor, ACE2.Adv Exp Med Biol. 2006;581:209-18. doi: 10.1007/978-0-387-33012-9_36. Adv Exp Med Biol. 2006. PMID: 17037532 Free PMC article. Review. No abstract available.
Cited by
-
Conformational Changes of the Receptor Binding Domain of SARS-CoV-2 Spike Protein and Prediction of a B-Cell Antigenic Epitope Using Structural Data.Front Artif Intell. 2021 Mar 25;4:630955. doi: 10.3389/frai.2021.630955. eCollection 2021. Front Artif Intell. 2021. PMID: 33842877 Free PMC article.
-
Development of animal models against emerging coronaviruses: From SARS to MERS coronavirus.Virology. 2015 May;479-480:247-58. doi: 10.1016/j.virol.2015.02.030. Epub 2015 Mar 16. Virology. 2015. PMID: 25791336 Free PMC article. Review.
-
Development of antibody resistance in emerging mutant strains of SARS CoV-2: Impediment for COVID-19 vaccines.Rev Med Virol. 2022 Sep;32(5):e2346. doi: 10.1002/rmv.2346. Epub 2022 Apr 13. Rev Med Virol. 2022. PMID: 35416390 Free PMC article. Review.
-
Phylogenetic perspectives on the epidemiology and origins of SARS and SARS-like coronaviruses.Infect Genet Evol. 2009 Dec;9(6):1185-96. doi: 10.1016/j.meegid.2009.09.015. Epub 2009 Sep 30. Infect Genet Evol. 2009. PMID: 19800030 Free PMC article. Review.
-
Molecular and Clinical Investigation of COVID-19: From Pathogenesis and Immune Responses to Novel Diagnosis and Treatment.Front Mol Biosci. 2022 May 19;9:770775. doi: 10.3389/fmolb.2022.770775. eCollection 2022. Front Mol Biosci. 2022. PMID: 35664675 Free PMC article. Review.
References
-
- Brunger, A. T., P. D. Adams, G. M. Clore, W. L. DeLano, P. Gros, R. W. Grosse-Kunstleve, J. S. Jiang, J. Kuszewski, M. Nilges, N. S. Pannu, R. J. Read, L. M. Rice, T. Simonson, and G. L. Warren. 1998. Crystallography & NMR system: a new software suite for macromolecular structure determination. Acta Crystallogr. D 54905-921. - PubMed
-
- Cowtan, K. 1994. Joint CCP4 and ESF-EACBM Newsl. Protein Crystallogr. 3134-38.
-
- Fenn, T. D., D. Ringe, and G. A. Petsko. 2003. POVScript+: a program for model and data visualization using persistence of vision ray-tracing. J. Appl. Crystallogr. 36944-947.
-
- Guan, Y., B. J. Zheng, Y. Q. He, X. L. Liu, Z. X. Zhuang, C. L. Cheung, S. W. Luo, P. H. Li, L. J. Zhang, Y. J. Guan, K. M. Butt, K. L. Wong, K. W. Chan, W. Lim, K. F. Shortridge, K. Y. Yuen, J. S. M. Peiris, and L. L. M. Poon. 2003. Isolation and characterization of viruses related to the SARS coronavirus from animals in Southern China. Science 302276-278. - PubMed
Publication types
MeSH terms
Substances
Grants and funding
LinkOut - more resources
Full Text Sources
Miscellaneous