Folding and assembly of large macromolecular complexes monitored by hydrogen-deuterium exchange and mass spectrometry
- PMID: 18394161
- PMCID: PMC2365927
- DOI: 10.1186/1475-2859-7-12
Folding and assembly of large macromolecular complexes monitored by hydrogen-deuterium exchange and mass spectrometry
Abstract
Recent advances in protein mass spectrometry (MS) have enabled determinations of hydrogen deuterium exchange (HDX) in large macromolecular complexes. HDX-MS became a valuable tool to follow protein folding, assembly and aggregation. The methodology has a wide range of applications in biotechnology ranging from quality control for over-expressed proteins and their complexes to screening of potential ligands and inhibitors. This review provides an introduction to protein folding and assembly followed by the principles of HDX and MS detection, and concludes with selected examples of applications that might be of interest to the biotechnology community.
Figures
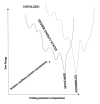
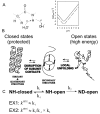
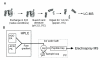
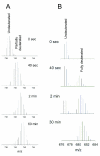
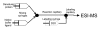
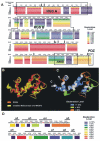
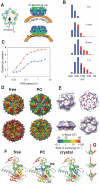
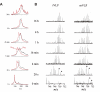
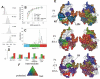
Similar articles
-
Hydrogen-Deuterium Exchange Coupled to Top- and Middle-Down Mass Spectrometry Reveals Histone Tail Dynamics before and after Nucleosome Assembly.Structure. 2018 Dec 4;26(12):1651-1663.e3. doi: 10.1016/j.str.2018.08.006. Epub 2018 Oct 4. Structure. 2018. PMID: 30293810 Free PMC article.
-
A Hydrogen-Deuterium Exchange Mass Spectrometry (HDX-MS) Platform for Investigating Peptide Biosynthetic Enzymes.J Vis Exp. 2020 May 4;(159). doi: 10.3791/61053. J Vis Exp. 2020. PMID: 32420996
-
Using Hydrogen-Deuterium Exchange Mass Spectrometry to Examine Protein-Membrane Interactions.Methods Enzymol. 2017;583:143-172. doi: 10.1016/bs.mie.2016.09.008. Epub 2016 Oct 18. Methods Enzymol. 2017. PMID: 28063489 Review.
-
Hydrogen deuterium exchange mass spectrometry applied to chaperones and chaperone-assisted protein folding.Expert Rev Proteomics. 2019 Jul;16(7):613-625. doi: 10.1080/14789450.2019.1633920. Epub 2019 Jun 24. Expert Rev Proteomics. 2019. PMID: 31215268 Review.
-
Online Hydrogen-Deuterium Exchange Traveling Wave Ion Mobility Mass Spectrometry (HDX-IM-MS): a Systematic Evaluation.J Am Soc Mass Spectrom. 2017 Jun;28(6):1192-1202. doi: 10.1007/s13361-017-1633-z. Epub 2017 Apr 3. J Am Soc Mass Spectrom. 2017. PMID: 28374315 Free PMC article.
Cited by
-
Characterizing Protein Structure, Dynamics and Conformation in Lyophilized Solids.Curr Pharm Des. 2015;21(40):5845-53. doi: 10.2174/1381612821666151008150735. Curr Pharm Des. 2015. PMID: 26446463 Free PMC article. Review.
-
Mapping the protein-protein interface between a toxin and its cognate antitoxin from the bacterial pathogen Streptococcus pyogenes.Biochemistry. 2011 May 17;50(19):4038-45. doi: 10.1021/bi200244k. Epub 2011 Apr 19. Biochemistry. 2011. PMID: 21466233 Free PMC article.
-
Two-stage, self-cycling process for the production of bacteriophages.Microb Cell Fact. 2010 Nov 1;9:81. doi: 10.1186/1475-2859-9-81. Microb Cell Fact. 2010. PMID: 21040541 Free PMC article.
-
Protein-peptide affinity determination using an h/d exchange dilution strategy: application to antigen-antibody interactions.J Am Soc Mass Spectrom. 2010 Oct;21(10):1660-7. doi: 10.1016/j.jasms.2010.03.030. Epub 2010 Mar 28. J Am Soc Mass Spectrom. 2010. PMID: 20444623 Free PMC article.
-
Estimating Constraints for Protection Factors from HDX-MS Data.Biophys J. 2019 Apr 2;116(7):1194-1203. doi: 10.1016/j.bpj.2019.02.024. Epub 2019 Mar 5. Biophys J. 2019. PMID: 30885379 Free PMC article.
References
LinkOut - more resources
Full Text Sources
Other Literature Sources