Novel interactions of ESCRT-III with LIP5 and VPS4 and their implications for ESCRT-III disassembly
- PMID: 18385515
- PMCID: PMC2397308
- DOI: 10.1091/mbc.e07-12-1263
Novel interactions of ESCRT-III with LIP5 and VPS4 and their implications for ESCRT-III disassembly
Abstract
The AAA+ ATPase VPS4 plays an essential role in multivesicular body biogenesis and is thought to act by disassembling ESCRT-III complexes. VPS4 oligomerization and ATPase activity are promoted by binding to LIP5. LIP5 also binds to the ESCRT-III like protein CHMP5/hVps60, but how this affects its function remains unclear. Here we confirm that LIP5 binds tightly to CHMP5, but also find that it binds well to additional ESCRT-III proteins including CHMP1B, CHMP2A/hVps2-1, and CHMP3/hVps24 but not CHMP4A/hSnf7-1 or CHMP6/hVps20. LIP5 binds to a different region within CHMP5 than within the other ESCRT-III proteins. In CHMP1B and CHMP2A, its binding site encompasses sequences at the proteins' extreme C-termini that overlap with "MIT interacting motifs" (MIMs) known to bind to VPS4. We find unexpected evidence of a second conserved binding site for VPS4 in CHMP2A and CHMP1B, suggesting that LIP5 and VPS4 may bind simultaneously to these proteins despite the overlap in their primary binding sites. Finally, LIP5 binds preferentially to soluble CHMP5 but instead to polymerized CHMP2A, suggesting that the newly defined interactions between LIP5 and ESCRT-III proteins may be regulated by ESCRT-III conformation. These studies point to a role for direct binding between LIP5 and ESCRT-III proteins that is likely to complement LIP5's previously described ability to regulate VPS4 activity.
Figures
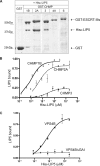
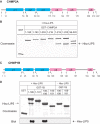
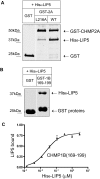
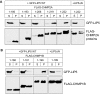
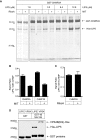
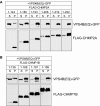
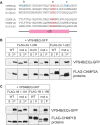
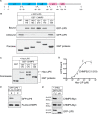
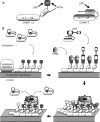
Comment in
- Mol Biol Cell. 19:2349.
Similar articles
-
Interactions of the human LIP5 regulatory protein with endosomal sorting complexes required for transport.J Biol Chem. 2012 Dec 21;287(52):43910-26. doi: 10.1074/jbc.M112.417899. Epub 2012 Oct 26. J Biol Chem. 2012. PMID: 23105106 Free PMC article.
-
A novel mechanism of regulating the ATPase VPS4 by its cofactor LIP5 and the endosomal sorting complex required for transport (ESCRT)-III protein CHMP5.J Biol Chem. 2015 Mar 13;290(11):7291-303. doi: 10.1074/jbc.M114.616730. Epub 2015 Jan 30. J Biol Chem. 2015. PMID: 25637630 Free PMC article.
-
Activation of human VPS4A by ESCRT-III proteins reveals ability of substrates to relieve enzyme autoinhibition.J Biol Chem. 2010 Nov 12;285(46):35428-38. doi: 10.1074/jbc.M110.126318. Epub 2010 Aug 30. J Biol Chem. 2010. PMID: 20805225 Free PMC article.
-
Meiotic Clade AAA ATPases: Protein Polymer Disassembly Machines.J Mol Biol. 2016 May 8;428(9 Pt B):1897-911. doi: 10.1016/j.jmb.2015.11.004. Epub 2015 Nov 10. J Mol Biol. 2016. PMID: 26555750 Free PMC article. Review.
-
Structures, Functions, and Dynamics of ESCRT-III/Vps4 Membrane Remodeling and Fission Complexes.Annu Rev Cell Dev Biol. 2018 Oct 6;34:85-109. doi: 10.1146/annurev-cellbio-100616-060600. Epub 2018 Aug 10. Annu Rev Cell Dev Biol. 2018. PMID: 30095293 Free PMC article. Review.
Cited by
-
Interactions of the human LIP5 regulatory protein with endosomal sorting complexes required for transport.J Biol Chem. 2012 Dec 21;287(52):43910-26. doi: 10.1074/jbc.M112.417899. Epub 2012 Oct 26. J Biol Chem. 2012. PMID: 23105106 Free PMC article.
-
Comprehensive analysis of the human ESCRT-III-MIT domain interactome reveals new cofactors for cytokinetic abscission.Elife. 2022 Sep 15;11:e77779. doi: 10.7554/eLife.77779. Elife. 2022. PMID: 36107470 Free PMC article.
-
Endocytosis and intracellular trafficking of ErbBs.Exp Cell Res. 2008 Oct 15;314(17):3093-106. doi: 10.1016/j.yexcr.2008.08.013. Epub 2008 Aug 28. Exp Cell Res. 2008. PMID: 18793634 Free PMC article. Review.
-
Characterization and Genetic Analyses of New Genes Coding for NOD2 Interacting Proteins.PLoS One. 2016 Nov 3;11(11):e0165420. doi: 10.1371/journal.pone.0165420. eCollection 2016. PLoS One. 2016. PMID: 27812135 Free PMC article.
-
The interferon-induced gene ISG15 blocks retrovirus release from cells late in the budding process.J Virol. 2010 May;84(9):4725-36. doi: 10.1128/JVI.02478-09. Epub 2010 Feb 17. J Virol. 2010. PMID: 20164219 Free PMC article.
References
-
- Azmi I. F., Davies B. A., Xiao J., Babst M., Xu Z., Katzmann D. J. ESCRT-III family members stimulate Vps4 ATPase activity directly or via Vta1. Dev. Cell. 2008;14:50–61. - PubMed
-
- Babst M. A protein's final ESCRT. Traffic. 2005;6:2–9. - PubMed
-
- Babst M., Katzmann D. J., Estepa-Sabal E. J., Meerloo T., Emr S. D. Escrt–III: an endosome-associated heterooligomeric protein complex required for mvb sorting. Dev. Cell. 2002;3:271–282. - PubMed
Publication types
MeSH terms
Substances
LinkOut - more resources
Full Text Sources
Molecular Biology Databases
Miscellaneous