Sphingomyelin synthases regulate production of diacylglycerol at the Golgi
- PMID: 18370930
- PMCID: PMC5125090
- DOI: 10.1042/BJ20071240
Sphingomyelin synthases regulate production of diacylglycerol at the Golgi
Abstract
SMS [SM (sphingomyelin) synthase] is a class of enzymes that produces SM by transferring a phosphocholine moiety on to ceramide. PC (phosphatidylcholine) is believed to be the phosphocholine donor of the reaction with consequent production of DAG (diacylglycerol), an important bioactive lipid. In the present study, by modulating SMS1 and SMS2 expression, the role of these enzymes on the elusive regulation of DAG was investigated. Because we found that modulation of SMS1 or SMS2 did not affect total levels of endogenous DAG in resting cells, whereas they produce DAG in vitro, the possibility that SMSs could modulate subcellular pools of DAG, once acute activation of the enzymes is triggered, was investigated. Stimulation of SM synthesis was induced by either treatment with short-chain ceramide analogues or by increasing endogenous ceramide at the plasma membrane, and a fluorescently labelled conventional C1 domain [from PKC (protein kinase C)] enhanced in its DAG binding activity was used to probe subcellular pools of DAG in the cell. With this approach, we found, using confocal microscopy and subcellular fractionation, that modulation of SMS1 and, to a lesser extent, SMS2 affected the formation of DAG at the Golgi apparatus. Similarly, down-regulation of SMS1 and SMS2 reduced the localization of the DAG-binding protein PKD (protein kinase D) to the Golgi. These results provide direct evidence that both enzymes are capable of regulating the formation of DAG in cells, that this pool of DAG is biologically active, and for the first time directly implicate SMS1 and SMS2 as regulators of DAG-binding proteins in the Golgi apparatus.
Figures
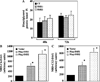
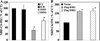
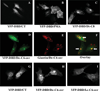
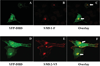
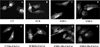
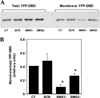
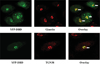
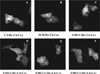
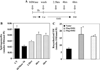
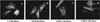
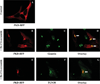
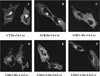
Similar articles
-
Sphingomyelin synthases regulate protein trafficking and secretion.PLoS One. 2011;6(9):e23644. doi: 10.1371/journal.pone.0023644. Epub 2011 Sep 27. PLoS One. 2011. PMID: 21980337 Free PMC article.
-
SMS overexpression and knockdown: impact on cellular sphingomyelin and diacylglycerol metabolism, and cell apoptosis.J Lipid Res. 2008 Feb;49(2):376-85. doi: 10.1194/jlr.M700401-JLR200. Epub 2007 Nov 2. J Lipid Res. 2008. PMID: 17982138
-
Inhibition of sphingomyelin synthase (SMS) affects intracellular sphingomyelin accumulation and plasma membrane lipid organization.Biochim Biophys Acta. 2007 Sep;1771(9):1186-94. doi: 10.1016/j.bbalip.2007.05.007. Epub 2007 Jun 6. Biochim Biophys Acta. 2007. PMID: 17616479 Free PMC article.
-
The sphingomyelin synthase family: proteins, diseases, and inhibitors.Biol Chem. 2017 Nov 27;398(12):1319-1325. doi: 10.1515/hsz-2017-0148. Biol Chem. 2017. PMID: 28742512 Review.
-
Role of ceramide/sphingomyelin (SM) balance regulated through "SM cycle" in cancer.Cell Signal. 2021 Nov;87:110119. doi: 10.1016/j.cellsig.2021.110119. Epub 2021 Aug 19. Cell Signal. 2021. PMID: 34418535 Review.
Cited by
-
Sphingomyelin metabolism at the plasma membrane: implications for bioactive sphingolipids.FEBS Lett. 2010 May 3;584(9):1887-94. doi: 10.1016/j.febslet.2009.10.058. Epub 2009 Oct 24. FEBS Lett. 2010. PMID: 19857494 Free PMC article. Review.
-
Role of sphingomyelin synthase in controlling the antimicrobial activity of neutrophils against Cryptococcus neoformans.PLoS One. 2010 Dec 28;5(12):e15587. doi: 10.1371/journal.pone.0015587. PLoS One. 2010. PMID: 21203393 Free PMC article.
-
Sphingolipid metabolic flow controls phosphoinositide turnover at the trans-Golgi network.EMBO J. 2017 Jun 14;36(12):1736-1754. doi: 10.15252/embj.201696048. Epub 2017 May 10. EMBO J. 2017. PMID: 28495678 Free PMC article.
-
GPRC5B-Mediated Sphingomyelin Synthase 2 Phosphorylation Plays a Critical Role in Insulin Resistance.iScience. 2018 Oct 26;8:250-266. doi: 10.1016/j.isci.2018.10.001. Epub 2018 Oct 6. iScience. 2018. PMID: 30343189 Free PMC article.
-
Deficiency of sphingomyelin synthase 1 but not sphingomyelin synthase 2 reduces bone formation due to impaired osteoblast differentiation.Mol Med. 2019 Dec 17;25(1):56. doi: 10.1186/s10020-019-0123-0. Mol Med. 2019. PMID: 31847800 Free PMC article.
References
-
- Ullman MDR, Radin N. The enzymatic formation of sphingomyelin from ceramide and lecithin in mouse liver. J. Biol. Chem. 1974;249:1506–1512. - PubMed
-
- Bernert JT, Ullman MD. Biosynthesis of sphingomyelin from erythro-ceramides and phosphatidylcholine by a microsomal cholinephosphoesterase. Biochim. Biophys. Acta. 1981;666:99–109. - PubMed
-
- Marggraf WD, Anderer FA, Kanfer J. The formation of sphingomyelin from phosphatidylcholine in plasma membrane preparations from mouse fibroblasts. Biochim. Biophys. Acta. 1981;664:61–73. - PubMed
-
- Marggraf WD, Zertani R, Anderer FA, Kanfer JN. The role of endogenous phosphatidylcholine and ceramide in the biosynthesis of sphingomyelin in mouse fibroblasts. Biochim. Biophys. Acta. 1982;710:314–323. - PubMed
-
- Voelker DRK, Kennedy EP. Cellular and enzymic synthesis of sphingomyelin. Biochemistry. 1982;21:2753–2759. - PubMed
Publication types
MeSH terms
Substances
Grants and funding
LinkOut - more resources
Full Text Sources
Other Literature Sources
Molecular Biology Databases