Compartment-specific transcription factors orchestrate angiogenesis gradients in the embryonic brain
- PMID: 18344991
- PMCID: PMC2754271
- DOI: 10.1038/nn2074
Compartment-specific transcription factors orchestrate angiogenesis gradients in the embryonic brain
Abstract
Prevailing notions of cerebral vascularization imply that blood vessels sprout passively into the brain parenchyma from pial vascular plexuses to meet metabolic needs of growing neuronal populations. Endothelial cells, building blocks of blood vessels, are thought to be homogeneous in the brain with respect to their origins, gene expression patterns and developmental mechanisms. These current notions that cerebral angiogenesis is regulated by local environmental signals contrast with current models of cell-autonomous regulation of neuronal development. Here we demonstrate that telencephalic angiogenesis in mice progresses in an orderly, ventral-to-dorsal gradient regulated by compartment-specific homeobox transcription factors. Our data offer new perspectives on intrinsic regulation of angiogenesis in the embryonic telencephalon, call for a revision of the current models of telencephalic angiogenesis and support novel roles for endothelial cells in brain development.
Figures
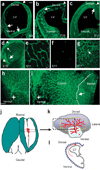
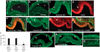
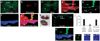
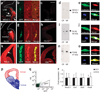
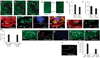
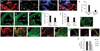
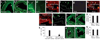
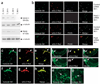
Similar articles
-
Normal ventral telencephalic expression of Pax6 is required for normal development of thalamocortical axons in embryonic mice.Neural Dev. 2009 Jun 5;4:19. doi: 10.1186/1749-8104-4-19. Neural Dev. 2009. PMID: 19500363 Free PMC article.
-
Removal of Pax6 partially rescues the loss of ventral structures in Shh null mice.Cereb Cortex. 2006 Jul;16 Suppl 1:i96-102. doi: 10.1093/cercor/bhk023. Cereb Cortex. 2006. PMID: 16766714
-
Dmrta1 regulates proneural gene expression downstream of Pax6 in the mammalian telencephalon.Genes Cells. 2013 Aug;18(8):636-49. doi: 10.1111/gtc.12061. Epub 2013 May 16. Genes Cells. 2013. PMID: 23679989
-
Pax6 is required at the telencephalic pallial-subpallial boundary for the generation of neuronal diversity in the postnatal limbic system.J Neurosci. 2011 Apr 6;31(14):5313-24. doi: 10.1523/JNEUROSCI.3867-10.2011. J Neurosci. 2011. PMID: 21471366 Free PMC article.
-
Loss- and gain-of-function analyses reveal targets of Pax6 in the developing mouse telencephalon.Mol Cell Neurosci. 2007 Jan;34(1):99-119. doi: 10.1016/j.mcn.2006.10.008. Epub 2006 Dec 8. Mol Cell Neurosci. 2007. PMID: 17158062
Cited by
-
Vascularisation of the central nervous system.Mech Dev. 2015 Nov;138 Pt 1:26-36. doi: 10.1016/j.mod.2015.07.001. Epub 2015 Jul 26. Mech Dev. 2015. PMID: 26222953 Free PMC article. Review.
-
Cerebrovascular defects in Foxc1 mutants correlate with aberrant WNT and VEGF-A pathways downstream of retinoic acid from the meninges.Dev Biol. 2016 Dec 1;420(1):148-165. doi: 10.1016/j.ydbio.2016.09.019. Epub 2016 Sep 23. Dev Biol. 2016. PMID: 27671872 Free PMC article.
-
Wnt-Dependent Oligodendroglial-Endothelial Interactions Regulate White Matter Vascularization and Attenuate Injury.Neuron. 2020 Dec 23;108(6):1130-1145.e5. doi: 10.1016/j.neuron.2020.09.033. Epub 2020 Oct 20. Neuron. 2020. PMID: 33086038 Free PMC article.
-
The cerebrofacial metameric syndromes: An embryological review and proposal of a novel classification scheme.Interv Neuroradiol. 2022 Oct;28(5):595-603. doi: 10.1177/15910199211044938. Epub 2021 Oct 19. Interv Neuroradiol. 2022. PMID: 34665049 Free PMC article. Review.
-
Perturbed neural activity disrupts cerebral angiogenesis during a postnatal critical period.Nature. 2014 Jan 16;505(7483):407-11. doi: 10.1038/nature12821. Epub 2013 Dec 4. Nature. 2014. PMID: 24305053 Free PMC article.
References
-
- Risau W. Mechanisms of angiogenesis. Nature. 1997;386:671–674. - PubMed
-
- Kurz H. Physiology of angiogenesis. J. Neurooncol. 2000;50:17–35. - PubMed
-
- Strong LH. The early embryonic pattern of internal vascularization of the mammalian cerebral cortex. J. Comp. Neurol. 1964;123:121–138. - PubMed
-
- Carmeliet P, Tessier-Lavigne M. Common mechanisms of nerve and blood vessel wiring. Nature. 2005;436:193–200. - PubMed
-
- Hogan KA, Ambler CA, Chapman DL, Bautch VL. The neural tube patterns vessels developmentally using the VEGF signaling pathway. Development. 2004;131:1503–1513. - PubMed
Publication types
MeSH terms
Substances
Grants and funding
LinkOut - more resources
Full Text Sources
Other Literature Sources
Research Materials
Miscellaneous