Sad3 and sad4 are required for saponin biosynthesis and root development in oat
- PMID: 18203919
- PMCID: PMC2254932
- DOI: 10.1105/tpc.107.056531
Sad3 and sad4 are required for saponin biosynthesis and root development in oat
Abstract
Avenacins are antimicrobial triterpene glycosides that are produced by oat (Avena) roots. These compounds confer broad-spectrum resistance to soil pathogens. Avenacin A-1, the major avenacin produced by oats, is strongly UV fluorescent and accumulates in root epidermal cells. We previously defined nine loci required for avenacin synthesis, eight of which are clustered. Mutants affected at seven of these (including Saponin-deficient1 [Sad1], the gene for the first committed enzyme in the pathway) have normal root morphology but reduced root fluorescence. In this study, we focus on mutations at the other two loci, Sad3 (also within the gene cluster) and Sad4 (unlinked), which result in stunted root growth, membrane trafficking defects in the root epidermis, and root hair deficiency. While sad3 and sad4 mutants both accumulate the same intermediate, monodeglucosyl avenacin A-1, the effect on avenacin A-1 glucosylation in sad4 mutants is only partial. sad1/sad1 sad3/sad3 and sad1/sad1 sad4/sad4 double mutants have normal root morphology, implying that the accumulation of incompletely glucosylated avenacin A-1 disrupts membrane trafficking and causes degeneration of the epidermis, with consequential effects on root hair formation. Various lines of evidence indicate that these effects are dosage-dependent. The significance of these data for the evolution and maintenance of the avenacin gene cluster is discussed.
Figures
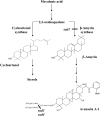
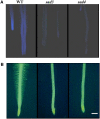
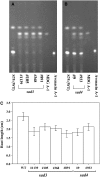
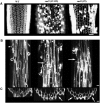
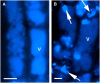
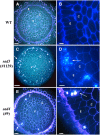
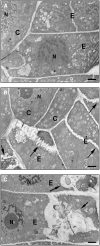
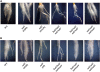
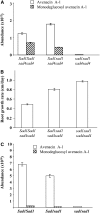
Similar articles
-
Investigation of triterpene synthesis and regulation in oats reveals a role for β-amyrin in determining root epidermal cell patterning.Proc Natl Acad Sci U S A. 2014 Jun 10;111(23):8679-84. doi: 10.1073/pnas.1401553111. Epub 2014 May 27. Proc Natl Acad Sci U S A. 2014. PMID: 24912185 Free PMC article.
-
Glycosyltransferases from oat (Avena) implicated in the acylation of avenacins.J Biol Chem. 2013 Feb 8;288(6):3696-704. doi: 10.1074/jbc.M112.426155. Epub 2012 Dec 20. J Biol Chem. 2013. PMID: 23258535 Free PMC article.
-
A different function for a member of an ancient and highly conserved cytochrome P450 family: from essential sterols to plant defense.Proc Natl Acad Sci U S A. 2006 Dec 5;103(49):18848-53. doi: 10.1073/pnas.0607849103. Epub 2006 Nov 21. Proc Natl Acad Sci U S A. 2006. PMID: 17124172 Free PMC article.
-
Evolution and genetics of root hair stripes in the root epidermis.J Exp Bot. 2001 Mar;52(Spec Issue):413-7. doi: 10.1093/jexbot/52.suppl_1.413. J Exp Bot. 2001. PMID: 11326047 Review.
-
Root hair development in grasses and cereals (Poaceae).Curr Opin Genet Dev. 2017 Aug;45:76-81. doi: 10.1016/j.gde.2017.03.009. Epub 2017 Apr 6. Curr Opin Genet Dev. 2017. PMID: 28391059 Review.
Cited by
-
Operons.Cell Mol Life Sci. 2009 Dec;66(23):3755-75. doi: 10.1007/s00018-009-0114-3. Epub 2009 Aug 7. Cell Mol Life Sci. 2009. PMID: 19662496 Free PMC article. Review.
-
Formation of plant metabolic gene clusters within dynamic chromosomal regions.Proc Natl Acad Sci U S A. 2011 Sep 20;108(38):16116-21. doi: 10.1073/pnas.1109273108. Epub 2011 Aug 29. Proc Natl Acad Sci U S A. 2011. PMID: 21876149 Free PMC article.
-
OSC2 and CYP716A14v2 catalyze the biosynthesis of triterpenoids for the cuticle of aerial organs of Artemisia annua.Plant Cell. 2015 Jan;27(1):286-301. doi: 10.1105/tpc.114.134486. Epub 2015 Jan 9. Plant Cell. 2015. PMID: 25576188 Free PMC article.
-
Terpenoid Transport in Plants: How Far from the Final Picture?Plants (Basel). 2023 Feb 1;12(3):634. doi: 10.3390/plants12030634. Plants (Basel). 2023. PMID: 36771716 Free PMC article. Review.
-
Plant terpenes that mediate below-ground interactions: prospects for bioengineering terpenoids for plant protection.Pest Manag Sci. 2019 Sep;75(9):2368-2377. doi: 10.1002/ps.5410. Epub 2019 Apr 8. Pest Manag Sci. 2019. PMID: 30884099 Free PMC article. Review.
References
-
- Bais, H.P., Weir, T.L., Perry, L.G., Gilroy, S., and Vivanco, J.M. (2006). The role of root exudates in rhizosphere interactions with plants and other organisms. Annu. Rev. Plant Biol. 57 233–266. - PubMed
-
- Bertin, C., Yang, X., and Weston, L.A. (2003). The role of root exudates and allelochemicals in the rhizosphere. Plant Soil 256 67–83.
-
- Bouarab, K., Melton, R., Peart, J., Baulcombe, D., and Osbourn, A. (2002). A saponin-detoxifying enzyme mediates suppression of plant defences. Nature 418 889–892. - PubMed
Publication types
MeSH terms
Substances
Associated data
- Actions
- Actions
Grants and funding
LinkOut - more resources
Full Text Sources
Other Literature Sources
Molecular Biology Databases