Evidence of compromised blood-spinal cord barrier in early and late symptomatic SOD1 mice modeling ALS
- PMID: 18030339
- PMCID: PMC2075163
- DOI: 10.1371/journal.pone.0001205
Evidence of compromised blood-spinal cord barrier in early and late symptomatic SOD1 mice modeling ALS
Abstract
Background: The blood-brain barrier (BBB), blood-spinal cord barrier (BSCB), and blood-cerebrospinal fluid barrier (BCSFB) control cerebral/spinal cord homeostasis by selective transport of molecules and cells from the systemic compartment. In the spinal cord and brain of both ALS patients and animal models, infiltration of T-cell lymphocytes, monocyte-derived macrophages and dendritic cells, and IgG deposits have been observed that may have a critical role in motor neuron damage. Additionally, increased levels of albumin and IgG have been found in the cerebrospinal fluid in ALS patients. These findings suggest altered barrier permeability in ALS. Recently, we showed disruption of the BBB and BSCB in areas of motor neuron degeneration in the brain and spinal cord in G93A SOD1 mice modeling ALS at both early and late stages of disease using electron microscopy. Examination of capillary ultrastructure revealed endothelial cell degeneration, which, along with astrocyte alteration, compromised the BBB and BSCB. However, the effect of these alterations upon barrier function in ALS is still unclear. The aim of this study was to determine the functional competence of the BSCB in G93A mice at different stages of disease.
Methodology/principal findings: Evans Blue (EB) dye was intravenously injected into ALS mice at early or late stage disease. Vascular leakage and the condition of basement membranes, endothelial cells, and astrocytes were investigated in cervical and lumbar spinal cords using immunohistochemistry. Results showed EB leakage in spinal cord microvessels from all G93A mice, indicating dysfunction in endothelia and basement membranes and confirming our previous ultrastructural findings on BSCB disruption. Additionally, downregulation of Glut-1 and CD146 expressions in the endothelial cells of the BSCB were found which may relate to vascular leakage.
Conclusions/significance: Results suggest that the BSCB is compromised in areas of motor neuron degeneration in ALS mice at both early and late stages of the disease.
Conflict of interest statement
Figures
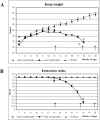
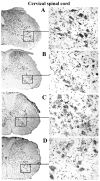
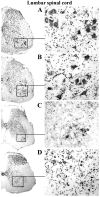
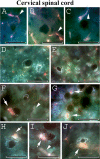
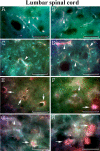
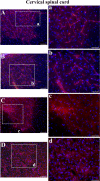
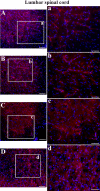
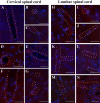
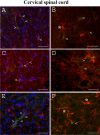
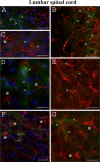
Similar articles
-
Transplantation of human bone marrow stem cells into symptomatic ALS mice enhances structural and functional blood-spinal cord barrier repair.Exp Neurol. 2018 Dec;310:33-47. doi: 10.1016/j.expneurol.2018.08.012. Epub 2018 Aug 30. Exp Neurol. 2018. PMID: 30172620 Free PMC article.
-
Ultrastructure of blood-brain barrier and blood-spinal cord barrier in SOD1 mice modeling ALS.Brain Res. 2007 Jul 9;1157:126-37. doi: 10.1016/j.brainres.2007.04.044. Epub 2007 Apr 24. Brain Res. 2007. PMID: 17512910
-
Blood-spinal cord barrier disruption contributes to early motor-neuron degeneration in ALS-model mice.Proc Natl Acad Sci U S A. 2014 Mar 18;111(11):E1035-42. doi: 10.1073/pnas.1401595111. Epub 2014 Mar 3. Proc Natl Acad Sci U S A. 2014. PMID: 24591593 Free PMC article.
-
CSF markers in amyotrophic lateral sclerosis.J Neural Transm (Vienna). 2012 Jul;119(7):747-57. doi: 10.1007/s00702-012-0806-y. Epub 2012 May 4. J Neural Transm (Vienna). 2012. PMID: 22555610 Review.
-
Altered blood-brain barrier and blood-spinal cord barrier dynamics in amyotrophic lateral sclerosis: Impact on medication efficacy and safety.Br J Pharmacol. 2022 Jun;179(11):2577-2588. doi: 10.1111/bph.15802. Epub 2022 Feb 7. Br J Pharmacol. 2022. PMID: 35048358 Review.
Cited by
-
Expression of the ALS-causing variant hSOD1(G93A) leads to an impaired integrity and altered regulation of claudin-5 expression in an in vitro blood-spinal cord barrier model.J Cereb Blood Flow Metab. 2015 Jul;35(7):1112-21. doi: 10.1038/jcbfm.2015.57. Epub 2015 Apr 8. J Cereb Blood Flow Metab. 2015. PMID: 25853911 Free PMC article.
-
T cell responses at diagnosis of amyotrophic lateral sclerosis predict disease progression.Nat Commun. 2022 Nov 8;13(1):6733. doi: 10.1038/s41467-022-34526-9. Nat Commun. 2022. PMID: 36347843 Free PMC article.
-
Astrocytic vesicle mobility in health and disease.Int J Mol Sci. 2013 May 27;14(6):11238-58. doi: 10.3390/ijms140611238. Int J Mol Sci. 2013. PMID: 23712361 Free PMC article. Review.
-
Activated protein C therapy slows ALS-like disease in mice by transcriptionally inhibiting SOD1 in motor neurons and microglia cells.J Clin Invest. 2009 Nov;119(11):3437-49. doi: 10.1172/JCI38476. Epub 2009 Oct 19. J Clin Invest. 2009. PMID: 19841542 Free PMC article.
-
Breached Barriers: A Scoping Review of Blood-Central Nervous System Barrier Pathology in Amyotrophic Lateral Sclerosis.Front Cell Neurosci. 2022 Mar 31;16:851563. doi: 10.3389/fncel.2022.851563. eCollection 2022. Front Cell Neurosci. 2022. PMID: 35431812 Free PMC article.
References
-
- Pardridge WM. Recent advances in blood-brain barrier transport. Ann Rev Pharmacol Toxicol. 1988;28:25–39. - PubMed
-
- Pardridge WM. Blood-brain barrier biology and methodology. J NeuroVirol. 1999;5:556–569. - PubMed
-
- Vorbrodt AW, Dobrogowska DH. Molecular anatomy of intercellular junctions in the brain endothelial and epithelial barriers: electron microscopist's view. Brain Res Rev. 2003;42:221–242. - PubMed
-
- Ballabh P, Braun A, Nedergaard M. The blood-brain barrier: an overview structure, regulation, and clinical implications. Neurobiol Dis. 2004;16:1–13. - PubMed
-
- Sharma HS. Pathophysiology of blood-spinal cord barrier in traumatic injury and repair. Curr Pharm Des. 2005;11:1353–1389. - PubMed
Publication types
MeSH terms
Substances
LinkOut - more resources
Full Text Sources
Other Literature Sources
Medical
Molecular Biology Databases
Miscellaneous