Distinct Dgrip84 isoforms correlate with distinct gamma-tubulins in Drosophila
- PMID: 18003974
- PMCID: PMC2174168
- DOI: 10.1091/mbc.e07-08-0801
Distinct Dgrip84 isoforms correlate with distinct gamma-tubulins in Drosophila
Abstract
Gamma-tubulin is an indispensable component of the animal centrosome and is required for proper microtubule organization. Within the cell, gamma-tubulin exists in a multiprotein complex containing between two (some yeasts) and six or more (metazoa) additional highly conserved proteins named gamma ring proteins (Grips) or gamma complex proteins (GCPs). gamma-Tubulin containing complexes isolated from Xenopus eggs or Drosophila embryos appear ring-shaped and have therefore been named the gamma-tubulin ring complex (gammaTuRC). Curiously, many organisms (including humans) have two distinct gamma-tubulin genes. In Drosophila, where the two gamma-tubulin isotypes have been studied most extensively, the gamma-tubulin genes are developmentally regulated: the "maternal" gamma-tubulin isotype (named gammaTub37CD according to its location on the genetic map) is expressed in the ovary and is deposited in the egg, where it is thought to orchestrate the meiotic and early embryonic cleavages. The second gamma-tubulin isotype (gammaTub23C) is ubiquitously expressed and persists in most of the cells of the adult fly. In those rare cases where both gamma-tubulins coexist in the same cell, they show distinct subcellular distributions and cell-cycle-dependent changes: gammaTub37CD mainly localizes to the centrosome, where its levels vary only slightly with the cell cycle. In contrast, the level of gammaTub23C at the centrosome increases at the beginning of mitosis, and gammaTub23C also associates with spindle pole microtubules. Here, we show that gammaTub23C forms discrete complexes that closely resemble the complexes formed by gammaTub37CD. Surprisingly, however, gammaTub23C associates with a distinct, longer splice variant of Dgrip84. This may reflect a role for Dgrip84 in regulating the activity and/or the location of the gamma-tubulin complexes formed with gammaTub37CD and gammaTub23C.
Figures
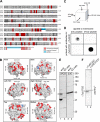
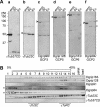
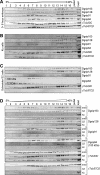
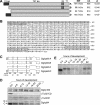
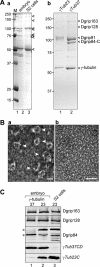
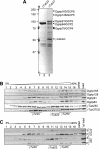
Similar articles
-
The Drosophila gamma-tubulin small complex subunit Dgrip84 is required for structural and functional integrity of the spindle apparatus.Mol Biol Cell. 2006 Jan;17(1):272-82. doi: 10.1091/mbc.e05-08-0722. Epub 2005 Oct 19. Mol Biol Cell. 2006. PMID: 16236791 Free PMC article.
-
Characterization and reconstitution of Drosophila gamma-tubulin ring complex subunits.J Cell Biol. 2000 Dec 25;151(7):1513-24. doi: 10.1083/jcb.151.7.1513. J Cell Biol. 2000. PMID: 11134079 Free PMC article.
-
Proper recruitment of gamma-tubulin and D-TACC/Msps to embryonic Drosophila centrosomes requires Centrosomin Motif 1.Mol Biol Cell. 2007 Oct;18(10):4037-49. doi: 10.1091/mbc.e07-05-0474. Epub 2007 Aug 1. Mol Biol Cell. 2007. PMID: 17671162 Free PMC article.
-
Gamma-tubulin at ten: progress and prospects.Cell Struct Funct. 1999 Oct;24(5):365-72. doi: 10.1247/csf.24.365. Cell Struct Funct. 1999. PMID: 15216894 Review.
-
Microtubule nucleation by γ-tubulin complexes.Nat Rev Mol Cell Biol. 2011 Oct 12;12(11):709-21. doi: 10.1038/nrm3209. Nat Rev Mol Cell Biol. 2011. PMID: 21993292 Free PMC article. Review.
Cited by
-
Microtubule polarity is instructive for many aspects of neuronal polarity.Dev Biol. 2022 Jun;486:56-70. doi: 10.1016/j.ydbio.2022.03.009. Epub 2022 Mar 25. Dev Biol. 2022. PMID: 35341730 Free PMC article.
-
Bilaterian Giant Ankyrins Have a Common Evolutionary Origin and Play a Conserved Role in Patterning the Axon Initial Segment.PLoS Genet. 2016 Dec 2;12(12):e1006457. doi: 10.1371/journal.pgen.1006457. eCollection 2016 Dec. PLoS Genet. 2016. PMID: 27911898 Free PMC article.
-
Γ-tubulin controls neuronal microtubule polarity independently of Golgi outposts.Mol Biol Cell. 2014 Jul 1;25(13):2039-50. doi: 10.1091/mbc.E13-09-0515. Epub 2014 May 7. Mol Biol Cell. 2014. PMID: 24807906 Free PMC article.
-
Kinesin-2 and Apc function at dendrite branch points to resolve microtubule collisions.Cytoskeleton (Hoboken). 2016 Jan;73(1):35-44. doi: 10.1002/cm.21270. Cytoskeleton (Hoboken). 2016. PMID: 26785384 Free PMC article.
-
Dendrite regeneration mediates functional recovery after complete dendrite removal.Dev Biol. 2023 May;497:18-25. doi: 10.1016/j.ydbio.2023.03.001. Epub 2023 Mar 3. Dev Biol. 2023. PMID: 36870669 Free PMC article.
References
-
- Andersen J. S., Wilkinson C. J., Mayor T., Mortensen P., Nigg E. A., Mann M. Proteomic characterization of the human centrosome by protein correlation profiling. Nature. 2003;426:570–574. - PubMed
-
- Aldaz H., Rice L. M., Stearns T., Agard D. A. Insights into microtubule nucleation from the crystal structure of human γ-tubulin. Nature. 2005;435:523–527. - PubMed
-
- Barbosa V., Gatt M., Rebollo E., Gonzalez C., Glover D. M. Drosophila dd4 mutants reveal that γTuRC is required to maintain juxtaposed half spindles in spermatocytes. J. Cell Sci. 2003;116:929–941. - PubMed
Publication types
MeSH terms
Substances
LinkOut - more resources
Full Text Sources
Molecular Biology Databases
Miscellaneous