An influenza virus replicon system in yeast identified Tat-SF1 as a stimulatory host factor for viral RNA synthesis
- PMID: 17991777
- PMCID: PMC2084326
- DOI: 10.1073/pnas.0705856104
An influenza virus replicon system in yeast identified Tat-SF1 as a stimulatory host factor for viral RNA synthesis
Abstract
Influenza viruses infect vertebrates, including mammals and birds. Influenza virus reverse-genetics systems facilitate the study of the structure and function of viral factors. In contrast, less is known about host factors involved in the replication process. Here, we developed a replication and transcription system of the negative-strand RNA genome of the influenza virus in Saccharomyces cerevisiae, which depends on viral RNAs, viral RNA polymerases, and nucleoprotein (NP). Disruption of SUB2 encoding an orthologue of human RAF-2p48/UAP56, a previously identified viral RNA synthesis stimulatory host factor, resulted in reduction of the viral RNA synthesis rate. Using a genome-wide set of yeast single-gene deletion strains, we found several host factor candidates affecting viral RNA synthesis. We found that among them, Tat-SF1, a mammalian homologue of yeast CUS2, was a stimulatory host factor in influenza virus RNA synthesis. Tat-SF1 interacted with free NP, but not with NP associated with RNA, and facilitated formation of RNA-NP complexes. These results suggest that Tat-SF1 may function as a molecular chaperone for NP, as does RAF-2p48/UAP56. This system has proven useful for further studies on the mechanism of influenza virus genome replication and transcription.
Conflict of interest statement
The authors declare no conflict of interest.
Figures
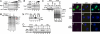
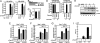
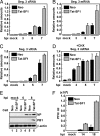
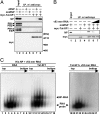
Similar articles
-
Cellular splicing factor RAF-2p48/NPI-5/BAT1/UAP56 interacts with the influenza virus nucleoprotein and enhances viral RNA synthesis.J Virol. 2001 Feb;75(4):1899-908. doi: 10.1128/JVI.75.4.1899-1908.2001. J Virol. 2001. PMID: 11160689 Free PMC article.
-
Pre-mRNA Processing Factor Prp18 Is a Stimulatory Factor of Influenza Virus RNA Synthesis and Possesses Nucleoprotein Chaperone Activity.J Virol. 2017 Jan 18;91(3):e01398-16. doi: 10.1128/JVI.01398-16. Print 2017 Feb 1. J Virol. 2017. PMID: 27852861 Free PMC article.
-
[Influenza virus replicon and host factors for its replication and transcription].Seikagaku. 2008 Dec;80(12):1128-33. Seikagaku. 2008. PMID: 19172795 Review. Japanese. No abstract available.
-
Replication-coupled and host factor-mediated encapsidation of the influenza virus genome by viral nucleoprotein.J Virol. 2011 Jul;85(13):6197-204. doi: 10.1128/JVI.00277-11. Epub 2011 Apr 20. J Virol. 2011. PMID: 21507964 Free PMC article.
-
Influenza virus RNA polymerase: insights into the mechanisms of viral RNA synthesis.Nat Rev Microbiol. 2016 Aug;14(8):479-93. doi: 10.1038/nrmicro.2016.87. Epub 2016 Jul 11. Nat Rev Microbiol. 2016. PMID: 27396566 Free PMC article. Review.
Cited by
-
Contribution of yeast models to virus research.Appl Microbiol Biotechnol. 2021 Jun;105(12):4855-4878. doi: 10.1007/s00253-021-11331-w. Epub 2021 Jun 4. Appl Microbiol Biotechnol. 2021. PMID: 34086116 Free PMC article. Review.
-
HMGB1 protein binds to influenza virus nucleoprotein and promotes viral replication.J Virol. 2012 Sep;86(17):9122-33. doi: 10.1128/JVI.00789-12. Epub 2012 Jun 13. J Virol. 2012. PMID: 22696656 Free PMC article.
-
Host- and strain-specific regulation of influenza virus polymerase activity by interacting cellular proteins.mBio. 2011 Aug 16;2(4):e00151-11. doi: 10.1128/mBio.00151-11. Print 2011. mBio. 2011. PMID: 21846828 Free PMC article.
-
Nuclear factor 90 negatively regulates influenza virus replication by interacting with viral nucleoprotein.J Virol. 2009 Aug;83(16):7850-61. doi: 10.1128/JVI.00735-09. Epub 2009 Jun 3. J Virol. 2009. PMID: 19494010 Free PMC article.
-
The intriguing phenomenon of cross-kingdom infections of plant and insect viruses to fungi: Can other animal viruses also cross-infect fungi?PLoS Pathog. 2023 Oct 26;19(10):e1011726. doi: 10.1371/journal.ppat.1011726. eCollection 2023 Oct. PLoS Pathog. 2023. PMID: 37883353 Free PMC article.
References
MeSH terms
Substances
LinkOut - more resources
Full Text Sources
Other Literature Sources
Molecular Biology Databases
Research Materials
Miscellaneous