Independent expression of synaptic and morphological plasticity associated with long-term depression
- PMID: 17989307
- PMCID: PMC6673249
- DOI: 10.1523/JNEUROSCI.2015-07.2007
Independent expression of synaptic and morphological plasticity associated with long-term depression
Abstract
Physiological and morphological alterations occur with long-term synaptic modifications, such as long-term potentiation (LTP) and long-term depression (LTD), but whether these two processes are independent or interactive is unclear. It is also unknown whether or how morphological modifications, like spine remodeling, may contribute to physiological modifications, such as trafficking of glutamate receptors which underlies, at least partially, the expression of LTP and LTD. In this study, we monitored spine size and synaptic responses simultaneously using combined two photon time-lapse imaging with patch-clamp recording in acute hippocampal slices. We show that spine shrinkage and LTD can occur independently of each other. We further show that changes in spine size are unrelated to trafficking of AMPA receptors (AMPARs) under various conditions: constitutive trafficking of AMPARs, insulin-induced internalization of AMPARs, or lateral movement of AMPARs to extrasynaptic sites. Induction of LTD of NMDA receptor-mediated responses (NMDAR-LTD) is associated with spine shrinkage. Nonetheless, NMDAR-LTD and spine shrinkage diverge in the downstream signaling events, and can occur independently of each other. Thus, spine shrinkage is not caused by or required for trafficking of glutamate receptors. In a broader sense, there is a clear dissociation between physiological and morphological expression of LTD. However, inhibition of actin depolymerization blocked the expression of LTD, suggesting that morphologically silent actin remodeling may be involved in the physiological expression of LTD and different subpopulations of actin filaments undergo changes during LTD.
Figures
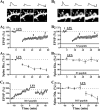
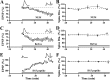
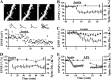
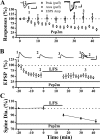
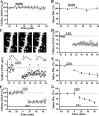
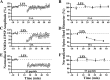
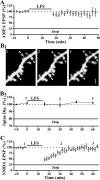
Similar articles
-
AMPA receptor subunit GluR1 (GluA1) serine-845 site is involved in synaptic depression but not in spine shrinkage associated with chemical long-term depression.J Neurophysiol. 2011 Apr;105(4):1897-907. doi: 10.1152/jn.00913.2010. Epub 2011 Feb 9. J Neurophysiol. 2011. PMID: 21307330 Free PMC article.
-
Distinct trafficking and expression mechanisms underlie LTP and LTD of NMDA receptor-mediated synaptic responses.Hippocampus. 2010 May;20(5):646-58. doi: 10.1002/hipo.20654. Hippocampus. 2010. PMID: 19489005
-
The N-methyl-D-aspartate receptor antagonist CPP alters synapse and spine structure and impairs long-term potentiation and long-term depression induced morphological plasticity in dentate gyrus of the awake rat.Neuroscience. 2010 Feb 17;165(4):1170-81. doi: 10.1016/j.neuroscience.2009.11.047. Epub 2009 Dec 1. Neuroscience. 2010. PMID: 19961908
-
LTD, LTP, and the sliding threshold for long-term synaptic plasticity.Hippocampus. 1996;6(1):35-42. doi: 10.1002/(SICI)1098-1063(1996)6:1<35::AID-HIPO7>3.0.CO;2-6. Hippocampus. 1996. PMID: 8878740 Review.
-
Regulation of neuronal PKA signaling through AKAP targeting dynamics.Eur J Cell Biol. 2006 Jul;85(7):627-33. doi: 10.1016/j.ejcb.2006.01.010. Epub 2006 Feb 28. Eur J Cell Biol. 2006. PMID: 16504338 Review.
Cited by
-
Persistence of coordinated long-term potentiation and dendritic spine enlargement at mature hippocampal CA1 synapses requires N-cadherin.J Neurosci. 2010 Jul 28;30(30):9984-9. doi: 10.1523/JNEUROSCI.1223-10.2010. J Neurosci. 2010. PMID: 20668183 Free PMC article.
-
Spine remodeling and synaptic modification.Mol Neurobiol. 2010 Feb;41(1):29-41. doi: 10.1007/s12035-009-8093-9. Epub 2010 Jan 6. Mol Neurobiol. 2010. PMID: 20049655 Review.
-
miR-191 and miR-135 are required for long-lasting spine remodelling associated with synaptic long-term depression.Nat Commun. 2014;5:3263. doi: 10.1038/ncomms4263. Nat Commun. 2014. PMID: 24535612 Free PMC article.
-
A stable proportion of Purkinje cell inputs from parallel fibers are silent during cerebellar maturation.Proc Natl Acad Sci U S A. 2021 Nov 9;118(45):e2024890118. doi: 10.1073/pnas.2024890118. Proc Natl Acad Sci U S A. 2021. PMID: 34740966 Free PMC article.
-
Forskolin reverses the O-GlcNAcylation dependent decrease in GABAAR current amplitude at hippocampal synapses possibly at a neurosteroid site on GABAARs.Res Sq [Preprint]. 2024 Apr 10:rs.3.rs-4140038. doi: 10.21203/rs.3.rs-4140038/v1. Res Sq. 2024. Update in: Sci Rep. 2024 Jul 29;14(1):17461. doi: 10.1038/s41598-024-66025-w PMID: 38659738 Free PMC article. Updated. Preprint.
References
-
- Beattie EC, Carroll RC, Yu X, Morishita W, Yasuda H, von Zastrow M, Malenka RC. Regulation of AMPA receptor endocytosis by a signaling mechanism shared with LTD. Nat Neurosci. 2000;3:1291–1300. - PubMed
-
- Borgdorff AJ, Choquet D. Regulation of AMPA receptor lateral movements. Nature. 2002;417:649–653. - PubMed
-
- Bredt DS, Nicoll RA. AMPA receptor trafficking at excitatory synapses. Neuron. 2003;40:361–379. - PubMed
Publication types
MeSH terms
LinkOut - more resources
Full Text Sources