Dominant TEL1-hy mutations compensate for Mec1 lack of functions in the DNA damage response
- PMID: 17954565
- PMCID: PMC2223299
- DOI: 10.1128/MCB.01214-07
Dominant TEL1-hy mutations compensate for Mec1 lack of functions in the DNA damage response
Abstract
Eukaryotic genome integrity is safeguarded by two highly conserved protein kinases that are called ATR and ATM for humans and Mec1 and Tel1 for Saccharomyces cerevisiae. Although they share sequence similarities and substrates, these protein kinases perform different specialized functions. In particular, Mec1 plays a key role in the DNA damage checkpoint response, whereas Tel1 primarily is involved in telomere homeostasis, and its checkpoint function is masked by the prevailing activity of Mec1. In order to understand how this specificity is achieved, we searched for TEL1 mutations able to compensate for the lack of Mec1 functions. Here, we describe seven independent dominant TEL1-hy alleles that are able to suppress, to different extents, both the hypersensitivity to genotoxic agents and the checkpoint defects of Mec1-deficient cells. Most of these alleles also cause telomere overelongation. In vitro kinase activity was increased compared to that of wild-type Tel1 in the Tel1-hy385, Tel1-hy394, Tel1-hy680, and Tel1-hy909 variants, but its activity was not affected by the TEL1-hy184 and TEL1-hy628 mutations and was slightly reduced by the TEL1-hy544 mutation. Thus, the phenotypes caused by at least some Tel1-hy variants are not simply the consequence of improved catalytic activity. Further characterization shows that Tel1-hy909 not only can sense and signal a single double-stranded DNA break, unlike wild-type Tel1, but also contributes more efficiently than Tel1 to single-stranded DNA accumulation at double-strand ends, thus enhancing Mec1 signaling activity. Moreover, it causes unscheduled checkpoint activation in unperturbed conditions and upregulates the checkpoint response to small amounts of DNA lesions. Finally, Tel1-hy544 can activate the checkpoint more efficiently than wild-type Tel1, while it causes telomere shortening, indicating that the checkpoint and telomeric functions of Tel1 can be separable.
Figures
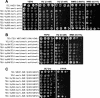
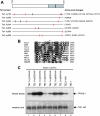
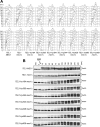
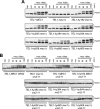
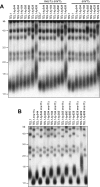
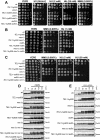
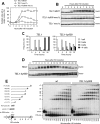
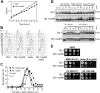
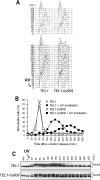
Similar articles
-
Requirement of the FATC domain of protein kinase Tel1 for localization to DNA ends and target protein recognition.Mol Biol Cell. 2015 Oct 1;26(19):3480-8. doi: 10.1091/mbc.E15-05-0259. Epub 2015 Aug 5. Mol Biol Cell. 2015. PMID: 26246601 Free PMC article.
-
Tel1/ATM Signaling to the Checkpoint Contributes to Replicative Senescence in the Absence of Telomerase.Genetics. 2019 Oct;213(2):411-429. doi: 10.1534/genetics.119.302391. Epub 2019 Aug 7. Genetics. 2019. PMID: 31391264 Free PMC article.
-
Cdc13 telomere capping decreases Mec1 association but does not affect Tel1 association with DNA ends.Mol Biol Cell. 2007 Jun;18(6):2026-36. doi: 10.1091/mbc.e06-12-1074. Epub 2007 Mar 21. Mol Biol Cell. 2007. PMID: 17377065 Free PMC article.
-
Interplays between ATM/Tel1 and ATR/Mec1 in sensing and signaling DNA double-strand breaks.DNA Repair (Amst). 2013 Oct;12(10):791-9. doi: 10.1016/j.dnarep.2013.07.009. Epub 2013 Aug 13. DNA Repair (Amst). 2013. PMID: 23953933 Review.
-
Budding yeast Rad51: a paradigm for how phosphorylation and intrinsic structural disorder regulate homologous recombination and protein homeostasis.Curr Genet. 2021 Jun;67(3):389-396. doi: 10.1007/s00294-020-01151-2. Epub 2021 Jan 12. Curr Genet. 2021. PMID: 33433732 Free PMC article. Review.
Cited by
-
Novel insights into the mechanism of cell cycle kinases Mec1(ATR) and Tel1(ATM).Crit Rev Biochem Mol Biol. 2021 Oct;56(5):441-454. doi: 10.1080/10409238.2021.1925218. Epub 2021 Jun 20. Crit Rev Biochem Mol Biol. 2021. PMID: 34151669 Free PMC article.
-
A balance between Tel1 and Rif2 activities regulates nucleolytic processing and elongation at telomeres.Mol Cell Biol. 2012 May;32(9):1604-17. doi: 10.1128/MCB.06547-11. Epub 2012 Feb 21. Mol Cell Biol. 2012. PMID: 22354991 Free PMC article.
-
Replication checkpoint: tuning and coordination of replication forks in s phase.Genes (Basel). 2013 Aug 19;4(3):388-434. doi: 10.3390/genes4030388. Genes (Basel). 2013. PMID: 24705211 Free PMC article.
-
DNA damage checkpoint and recombinational repair differentially affect the replication stress tolerance of Smc6 mutants.Mol Biol Cell. 2013 Aug;24(15):2431-41. doi: 10.1091/mbc.E12-11-0836. Epub 2013 Jun 19. Mol Biol Cell. 2013. PMID: 23783034 Free PMC article.
-
The DNA damage checkpoint: A tale from budding yeast.Front Genet. 2022 Sep 15;13:995163. doi: 10.3389/fgene.2022.995163. eCollection 2022. Front Genet. 2022. PMID: 36186482 Free PMC article. Review.
References
-
- Alcasabas, A. A., A. J. Osborn, J. Bachant, F. Hu, P. J. Werler, K. Bousset, K. Furuya, J. F. Diffley, A. M. Carr, and S. J. Elledge. 2001. Mrc1 transduces signals of DNA replication stress to activate Rad53. Nat. Cell Biol. 3958-965. - PubMed
-
- Bakkenist, C. J., and M. B. Kastan. 2003. DNA damage activates ATM through intermolecular autophosphorylation and dimer dissociation. Nature 421499-506. - PubMed
-
- Bi, X., S. C. Wei, and Y. S. Rong. 2004. Telomere protection without a telomerase; the role of ATM and Mre11 in Drosophila telomere maintenance. Curr. Biol. 141348-1353. - PubMed
-
- Chakhparonian, M., D. Faucher, and R. J. Wellinger. 2005. A mutation in yeast Tel1p that causes differential effects on the DNA damage checkpoint and telomere maintenance. Curr. Genet. 48310-322. - PubMed
Publication types
MeSH terms
Substances
LinkOut - more resources
Full Text Sources
Molecular Biology Databases
Research Materials
Miscellaneous