The development of synaptic plasticity induction rules and the requirement for postsynaptic spikes in rat hippocampal CA1 pyramidal neurones
- PMID: 17932146
- PMCID: PMC2375477
- DOI: 10.1113/jphysiol.2007.142984
The development of synaptic plasticity induction rules and the requirement for postsynaptic spikes in rat hippocampal CA1 pyramidal neurones
Abstract
Coincident pre- and postsynaptic activity induces synaptic plasticity at the Schaffer collateral synapse onto CA1 pyramidal neurones. The precise timing, frequency and number of coincident action potentials required to induce synaptic plasticity is currently unknown. In this study we show that the postsynaptic activity required for the induction of long-term potentiation (LTP) changes with development. In acute slices from adult rats, coincident pre- and postsynaptic theta burst stimulation (TBS) induced LTP and we show that multiple high-frequency postsynaptic spikes are required. In contrast, in acute slices from juvenile (P14) rats, TBS failed to induce LTP unless the excitatory postsynaptic potentials (EPSPs) were of sufficient magnitude to initiate action potentials. We also show that coincident individual pre- and postsynaptic action potentials are only capable of inducing LTP in the juvenile when given at a frequency greater than 5 Hz and that the timing of individual pre- and postsynaptic action potentials relative to one another is not important. Finally, we show that local tetrodotoxin (TTX) application to the soma blocked LTP in adults, but not juveniles. These data demonstrate that somatic spiking is more important for LTP induction in the adult as opposed to juvenile rats and we hypothesize that the basis for this is the ability of action potentials in the postsynaptic CA1 pyramidal neurone to back-propagate into the dendrites. Therefore, the pre- and postsynaptic activity patterns required to induce LTP mature as the hippocampus develops.
Figures
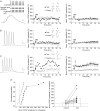
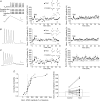
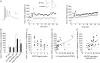
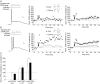
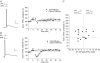
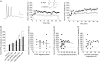
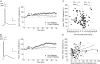
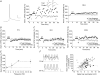
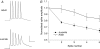
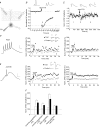
Similar articles
-
Long-term population spike-timing-dependent plasticity promotes synaptic tagging but not cross-tagging in rat hippocampal area CA1.Proc Natl Acad Sci U S A. 2019 Mar 19;116(12):5737-5746. doi: 10.1073/pnas.1817643116. Epub 2019 Feb 28. Proc Natl Acad Sci U S A. 2019. PMID: 30819889 Free PMC article.
-
Long-term plasticity at excitatory synapses on aspinous interneurons in area CA1 lacks synaptic specificity.J Neurophysiol. 1998 Jan;79(1):13-20. doi: 10.1152/jn.1998.79.1.13. J Neurophysiol. 1998. PMID: 9425172
-
Postsynaptic complex spike bursting enables the induction of LTP by theta frequency synaptic stimulation.J Neurosci. 1998 Sep 15;18(18):7118-26. doi: 10.1523/JNEUROSCI.18-18-07118.1998. J Neurosci. 1998. PMID: 9736635 Free PMC article.
-
Natural patterns of activity and long-term synaptic plasticity.Curr Opin Neurobiol. 2000 Apr;10(2):172-9. doi: 10.1016/s0959-4388(00)00076-3. Curr Opin Neurobiol. 2000. PMID: 10753798 Free PMC article. Review.
-
Theta-burst LTP.Brain Res. 2015 Sep 24;1621:38-50. doi: 10.1016/j.brainres.2014.10.034. Epub 2014 Oct 27. Brain Res. 2015. PMID: 25452022 Free PMC article. Review.
Cited by
-
Mossy fiber-evoked subthreshold responses induce timing-dependent plasticity at hippocampal CA3 recurrent synapses.Proc Natl Acad Sci U S A. 2014 Mar 18;111(11):4303-8. doi: 10.1073/pnas.1317667111. Epub 2014 Feb 18. Proc Natl Acad Sci U S A. 2014. PMID: 24550458 Free PMC article.
-
Multiple forms of metaplasticity at a single hippocampal synapse during late postnatal development.Dev Cogn Neurosci. 2015 Apr;12:145-54. doi: 10.1016/j.dcn.2015.01.009. Epub 2015 Feb 19. Dev Cogn Neurosci. 2015. PMID: 25752732 Free PMC article. Review.
-
A Ca-Based Computational Model for NMDA Receptor-Dependent Synaptic Plasticity at Individual Post-Synaptic Spines in the Hippocampus.Front Synaptic Neurosci. 2010 Jul 21;2:31. doi: 10.3389/fnsyn.2010.00031. eCollection 2010. Front Synaptic Neurosci. 2010. PMID: 21423517 Free PMC article.
-
Synaptic depolarization is more effective than back-propagating action potentials during induction of associative long-term potentiation in hippocampal pyramidal neurons.J Neurosci. 2009 Mar 11;29(10):3233-41. doi: 10.1523/JNEUROSCI.6000-08.2009. J Neurosci. 2009. PMID: 19279260 Free PMC article.
-
Ripples make waves: binding structured activity and plasticity in hippocampal networks.Neural Plast. 2011;2011:960389. doi: 10.1155/2011/960389. Epub 2011 Sep 27. Neural Plast. 2011. PMID: 21961073 Free PMC article. Review.
References
-
- Barria A, Malinow R. Subunit-specific NMDA receptor trafficking to synapses. Neuron. 2002;35:345–353. - PubMed
-
- Bliss TVP, Collingridge GL. A synaptic model of memory – long-term potentiation in the hippocampus. Nature. 1993;361:31–39. - PubMed
-
- Callaway JC, Ross WN. Frequency-dependent propagation of sodium action-potentials in dendrites of hippocampal CA1 pyramidal neurons. J Neurophysiol. 1995;74:1395–1403. - PubMed
Publication types
MeSH terms
Grants and funding
LinkOut - more resources
Full Text Sources
Research Materials
Miscellaneous