Tissue-specific expression of betaKlotho and fibroblast growth factor (FGF) receptor isoforms determines metabolic activity of FGF19 and FGF21
- PMID: 17623664
- PMCID: PMC2496965
- DOI: 10.1074/jbc.M704165200
Tissue-specific expression of betaKlotho and fibroblast growth factor (FGF) receptor isoforms determines metabolic activity of FGF19 and FGF21
Abstract
The fibroblast growth factor (FGF) 19 subfamily of ligands, FGF19, FGF21, and FGF23, function as hormones that regulate bile acid, fatty acid, glucose, and phosphate metabolism in target organs through activating FGF receptors (FGFR1-4). We demonstrated that Klotho and betaKlotho, homologous single-pass transmembrane proteins that bind to FGFRs, are required for metabolic activity of FGF23 and FGF21, respectively. Here we show that, like FGF21, FGF19 also requires betaKlotho. Both FGF19 and FGF21 can signal through FGFR1-3 bound by betaKlotho and increase glucose uptake in adipocytes expressing FGFR1. Additionally, both FGF19 and FGF21 bind to the betaKlotho-FGFR4 complex; however, only FGF19 signals efficiently through FGFR4. Accordingly, FGF19, but not FGF21, activates FGF signaling in hepatocytes that primarily express FGFR4 and reduces transcription of CYP7A1 that encodes the rate-limiting enzyme for bile acid synthesis. We conclude that the expression of betaKlotho, in combination with particular FGFR isoforms, determines the tissue-specific metabolic activities of FGF19 and FGF21.
Figures

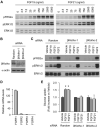
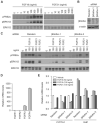
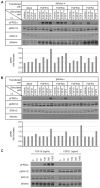
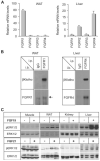
Similar articles
-
betaKlotho is required for fibroblast growth factor (FGF) 21 signaling through FGF receptor (FGFR) 1c and FGFR3c.Mol Endocrinol. 2008 Apr;22(4):1006-14. doi: 10.1210/me.2007-0313. Epub 2008 Jan 10. Mol Endocrinol. 2008. PMID: 18187602 Free PMC article.
-
C-terminal tail of FGF19 determines its specificity toward Klotho co-receptors.J Biol Chem. 2008 Nov 28;283(48):33304-9. doi: 10.1074/jbc.M803319200. Epub 2008 Oct 1. J Biol Chem. 2008. PMID: 18829467 Free PMC article.
-
Differential specificity of endocrine FGF19 and FGF21 to FGFR1 and FGFR4 in complex with KLB.PLoS One. 2012;7(3):e33870. doi: 10.1371/journal.pone.0033870. Epub 2012 Mar 19. PLoS One. 2012. PMID: 22442730 Free PMC article.
-
The Klotho gene family and the endocrine fibroblast growth factors.Curr Opin Nephrol Hypertens. 2008 Jul;17(4):368-72. doi: 10.1097/MNH.0b013e3282ffd994. Curr Opin Nephrol Hypertens. 2008. PMID: 18660672 Review.
-
The structural biology of the FGF19 subfamily.Adv Exp Med Biol. 2012;728:1-24. doi: 10.1007/978-1-4614-0887-1_1. Adv Exp Med Biol. 2012. PMID: 22396159 Free PMC article. Review.
Cited by
-
Fibroblast growth factor 21 in heart failure.Heart Fail Rev. 2023 Jan;28(1):261-272. doi: 10.1007/s10741-022-10268-0. Epub 2022 Aug 27. Heart Fail Rev. 2023. PMID: 36028609 Free PMC article. Review.
-
FGF21 regulates hepatic metabolic pathways to improve steatosis and inflammation.Endocr Connect. 2020 Aug;9(8):755-768. doi: 10.1530/EC-20-0152. Endocr Connect. 2020. PMID: 32688339 Free PMC article.
-
Non-alcoholic fatty liver disease and liver secretome.Arch Pharm Res. 2022 Dec;45(12):938-963. doi: 10.1007/s12272-022-01419-w. Epub 2022 Nov 28. Arch Pharm Res. 2022. PMID: 36441472 Free PMC article. Review.
-
The Role of the FGF19 Family in the Pathogenesis of Gestational Diabetes: A Narrative Review.Int J Mol Sci. 2023 Dec 9;24(24):17298. doi: 10.3390/ijms242417298. Int J Mol Sci. 2023. PMID: 38139126 Free PMC article. Review.
-
Klotho in Cancer: Potential Diagnostic and Prognostic Applications.Diagnostics (Basel). 2023 Oct 31;13(21):3357. doi: 10.3390/diagnostics13213357. Diagnostics (Basel). 2023. PMID: 37958253 Free PMC article. Review.
References
-
- Itoh N, Ornitz DM. Trends Genet. 2004;20:563–569. - PubMed
-
- Inagaki T, Choi M, Moschetta A, Peng L, Cummins CL, McDonald JG, Luo G, Jones SA, Goodwin B, Richardson JA, Gerard RD, Repa JJ, Mangelsdorf DJ, Kliewer SA. Cell Metab. 2005;2:217–225. - PubMed
-
- Choi M, Moschetta A, Bookout AL, Peng L, Umetani M, Holmstrom SR, Suino-Powell K, Xu HE, Richardson JA, Gerard RD, Mangelsdorf DJ, Kliewer SA. Nat. Med. 2006;12:1253–1255. - PubMed
-
- Inagaki T, Dutchak P, Zhao G, Ding X, Gautron L, Parameswara V, Li Y, Goetz R, Mohammadi M, Esser V, Elmquist JK, Gerard RD, Burgess SC, Hammer RE, Mangelsdorf DJ, Kliewer SA. Cell Metab. 2007;5:415–425. - PubMed
-
- Badman MK, Pissios P, Kennedy AR, Koukos G, Flier JS, Maratos-Flier E. Cell Metab. 2007;5:426–437. - PubMed
Publication types
MeSH terms
Substances
Grants and funding
LinkOut - more resources
Full Text Sources
Other Literature Sources
Molecular Biology Databases
Miscellaneous