Modulation of TASK-like background potassium channels in rat arterial chemoreceptor cells by intracellular ATP and other nucleotides
- PMID: 17615104
- PMCID: PMC2156202
- DOI: 10.1113/jphysiol.2007.135657
Modulation of TASK-like background potassium channels in rat arterial chemoreceptor cells by intracellular ATP and other nucleotides
Abstract
The carotid body's physiological role is to sense arterial oxygen, CO(2) and pH. It is however, also powerfully excited by inhibitors of oxidative phosphorylation. This latter observation is the cornerstone of the mitochondrial hypothesis which proposes that oxygen is sensed through changes in energy metabolism. All of these stimuli act in a similar manner, i.e. by inhibiting a background TASK-like potassium channel (K(B)) they induce membrane depolarization and thus neurosecretion. In this study we have evaluated the role of ATP in modulating K(B) channels. We find that K(B) channels are strongly activated by MgATP (but not ATP(4)(-)) within the physiological range (K(1/2) = 2.3 mm). This effect was mimicked by other Mg-nucleotides including GTP, UTP, AMP-PCP and ATP-gamma-S, but not by PP(i) or AMP, suggesting that channel activity is regulated by a Mg-nucleotide sensor. Channel activation by MgATP was not antagonized by either 1 mm AMP or 500 microm ADP. Thus MgATP is probably the principal nucleotide regulating channel activity in the intact cell. We therefore investigated the effects of metabolic inhibition upon both [Mg(2+)](i), as an index of MgATP depletion, and channel activity in cell-attached patches. The extent of increase in [Mg(2+)](i) (and thus MgATP depletion) in response to inhibition of oxidative phosphorylation were consistent with a decline in [MgATP](i) playing a prominent role in mediating inhibition of K(B) channel activity, and the response of arterial chemoreceptors to metabolic compromise.
Figures
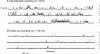
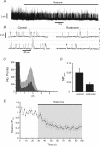
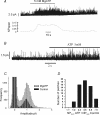
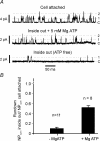
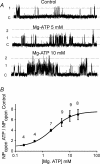
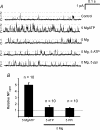
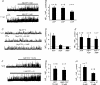
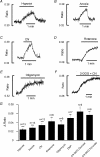
Similar articles
-
Dual actions of the metabolic inhibitor, sodium azide on K(ATP) channel currents in the rat CRI-G1 insulinoma cell line.Br J Pharmacol. 1999 Jan;126(1):51-60. doi: 10.1038/sj.bjp.0702267. Br J Pharmacol. 1999. PMID: 10051120 Free PMC article.
-
Muscarinic modulation of TASK-like background potassium channel in rat carotid body chemoreceptor cells.Brain Res. 2010 Apr 6;1323:74-83. doi: 10.1016/j.brainres.2010.01.091. Epub 2010 Feb 11. Brain Res. 2010. PMID: 20153302
-
Biophysical properties and metabolic regulation of a TASK-like potassium channel in rat carotid body type 1 cells.Am J Physiol Lung Cell Mol Physiol. 2004 Jan;286(1):L221-30. doi: 10.1152/ajplung.00010.2003. Epub 2003 Sep 22. Am J Physiol Lung Cell Mol Physiol. 2004. PMID: 14504065
-
TASK channels in arterial chemoreceptors and their role in oxygen and acid sensing.Pflugers Arch. 2015 May;467(5):1013-25. doi: 10.1007/s00424-015-1689-1. Epub 2015 Jan 28. Pflugers Arch. 2015. PMID: 25623783 Free PMC article. Review.
-
Cellular distribution of oxygen sensor candidates-oxidases, cytochromes, K+-channels--in the carotid body.Microsc Res Tech. 2002 Nov 1;59(3):234-42. doi: 10.1002/jemt.10197. Microsc Res Tech. 2002. PMID: 12384967 Review.
Cited by
-
Oxygen sensitivity of mitochondrial function in rat arterial chemoreceptor cells.J Physiol. 2013 Jul 15;591(14):3549-63. doi: 10.1113/jphysiol.2013.257741. Epub 2013 May 13. J Physiol. 2013. PMID: 23671162 Free PMC article.
-
Peripheral chemoreceptors: function and plasticity of the carotid body.Compr Physiol. 2012 Jan;2(1):141-219. doi: 10.1002/cphy.c100069. Compr Physiol. 2012. PMID: 23728973 Free PMC article. Review.
-
Characterization of an ATP-sensitive K(+) channel in rat carotid body glomus cells.Respir Physiol Neurobiol. 2011 Aug 15;177(3):247-55. doi: 10.1016/j.resp.2011.04.015. Epub 2011 Apr 22. Respir Physiol Neurobiol. 2011. PMID: 21536154 Free PMC article.
-
Gasotransmitter regulation of ion channels: a key step in O2 sensing by the carotid body.Physiology (Bethesda). 2014 Jan;29(1):49-57. doi: 10.1152/physiol.00034.2013. Physiology (Bethesda). 2014. PMID: 24382871 Free PMC article. Review.
-
Non-additive interactions between mitochondrial complex IV blockers and hypoxia in rat carotid body responses.Respir Physiol Neurobiol. 2014 Jan 1;190:62-9. doi: 10.1016/j.resp.2013.09.009. Epub 2013 Oct 2. Respir Physiol Neurobiol. 2014. PMID: 24096081 Free PMC article.
References
-
- Abraham MR, Selivanov VA, Hodgson DM, Pucar D, Zingman LV, Wieringa B, Dzeja PP, Alekseev AE, Terzic A. Coupling of cell energetics with membrane metabolic sensing. Integrative signaling through creatine kinase phosphotransfer disrupted by M-CK gene knock-out. J Biol Chem. 2002;277:24427–24434. - PubMed
-
- Anichkov S, Belen'kii M. Pharmacology of the Carotid Body Chemoreceptors. Oxford: Pergamon Press; 1963.
-
- Ashcroft FM. Adenosine 5′-triphosphate-sensitive potassium channels. Annu Rev Neurosci. 1988;11:97–118. - PubMed
-
- Bayliss DA, Talley EM, Sirois JE, Lei Q. TASK-1 is a highly modulated pH-sensitive ‘leak’ K+ channel expressed in brainstem respiratory neurons. Respir Physiol. 2001;129:159–174. - PubMed
Publication types
MeSH terms
Substances
LinkOut - more resources
Full Text Sources
Medical
Miscellaneous