DCL4 targets Cucumber mosaic virus satellite RNA at novel secondary structures
- PMID: 17609283
- PMCID: PMC1951434
- DOI: 10.1128/JVI.02885-06
DCL4 targets Cucumber mosaic virus satellite RNA at novel secondary structures
Abstract
It has been reported that plant virus-derived small interfering RNAs (vsiRNAs) originated predominantly from structured single-stranded viral RNA of a positive single-stranded RNA virus replicating in the cytoplasm and from the nuclear stem-loop 35S leader RNA of a double-stranded DNA (dsDNA) virus. Increasing lines of evidence have also shown that hierarchical actions of plant Dicer-like (DCL) proteins are required in the biogenesis process of small RNAs, and DCL4 is the primary producer of vsiRNAs. However, the structures of such single-stranded viral RNA that can be recognized by DCLs remain unknown. In an attempt to determine these structures, we have cloned siRNAs derived from the satellite RNA (satRNA) of Cucumber mosaic virus (CMV-satRNA) and studied the relationship between satRNA-derived siRNAs (satsiRNAs) and satRNA secondary structure. satsiRNAs were confirmed to be derived from single-stranded satRNA and are primarily 21 (64.7%) or 22 (22%) nucleotides (nt) in length. The most frequently cloned positive-strand satsiRNAs were found to derive from novel hairpins that differ from the structure of known DCL substrates, miRNA and siRNA precursors, which are prevalent stem-loop-shaped or dsRNAs. DCL4 was shown to be the primary producer of satsiRNAs. In the absence of DCL4, only 22-nt satsiRNAs were detected. Our results suggest that DCL4 is capable of accessing flexibly structured single-stranded RNA substrates (preferably T-shaped hairpins) to produce satsiRNAs. This result reveals that viral RNA of diverse structures may stimulate antiviral DCL activities in plant cells.
Figures
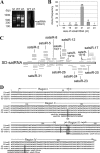
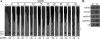
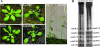
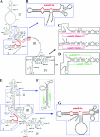
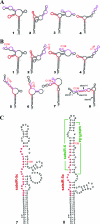
Similar articles
-
Cloning and profiling of small RNAs from cucumber mosaic virus satellite RNA.Methods Mol Biol. 2015;1236:99-109. doi: 10.1007/978-1-4939-1743-3_9. Methods Mol Biol. 2015. PMID: 25287499
-
Global analyses of small interfering RNAs derived from Bamboo mosaic virus and its associated satellite RNAs in different plants.PLoS One. 2010 Aug 2;5(8):e11928. doi: 10.1371/journal.pone.0011928. PLoS One. 2010. PMID: 20689857 Free PMC article.
-
Repair of the 3' proximal and internal deletions of a satellite RNA associated with Cucumber mosaic virus is directed toward restoring structural integrity.Virology. 2014 Feb;450-451:222-32. doi: 10.1016/j.virol.2013.12.008. Epub 2014 Jan 7. Virology. 2014. PMID: 24503085
-
Plant dicer-like proteins: double-stranded RNA-cleaving enzymes for small RNA biogenesis.J Plant Res. 2017 Jan;130(1):33-44. doi: 10.1007/s10265-016-0877-1. Epub 2016 Nov 24. J Plant Res. 2017. PMID: 27885504 Review.
-
Satellite RNAs and Satellite Viruses.Mol Plant Microbe Interact. 2016 Mar;29(3):181-6. doi: 10.1094/MPMI-10-15-0232-FI. Epub 2016 Feb 3. Mol Plant Microbe Interact. 2016. PMID: 26551994 Review.
Cited by
-
Differential expression of tomato spotted wilt virus-derived viral small RNAs in infected commercial and experimental host plants.PLoS One. 2013 Oct 15;8(10):e76276. doi: 10.1371/journal.pone.0076276. eCollection 2013. PLoS One. 2013. PMID: 24143182 Free PMC article.
-
A conserved RNA structure is essential for a satellite RNA-mediated inhibition of helper virus accumulation.Nucleic Acids Res. 2019 Sep 5;47(15):8255-8271. doi: 10.1093/nar/gkz564. Nucleic Acids Res. 2019. PMID: 31269212 Free PMC article.
-
Biochemical requirements for two Dicer-like activities from wheat germ.PLoS One. 2015 Jan 23;10(1):e0116736. doi: 10.1371/journal.pone.0116736. eCollection 2015. PLoS One. 2015. PMID: 25615604 Free PMC article.
-
Interfering Satellite RNAs of Bamboo mosaic virus.Front Microbiol. 2017 May 4;8:787. doi: 10.3389/fmicb.2017.00787. eCollection 2017. Front Microbiol. 2017. PMID: 28522996 Free PMC article. Review.
-
Diverse Functions of Small RNAs in Different Plant-Pathogen Communications.Front Microbiol. 2016 Oct 4;7:1552. doi: 10.3389/fmicb.2016.01552. eCollection 2016. Front Microbiol. 2016. PMID: 27757103 Free PMC article. Review.
References
-
- Baulcombe, D. 2004. RNA silencing in plants. Nature 431:356-363. - PubMed
Publication types
MeSH terms
Substances
LinkOut - more resources
Full Text Sources
Molecular Biology Databases
Research Materials