Immunogenicity of influenza virus vaccine is increased by anti-gal-mediated targeting to antigen-presenting cells
- PMID: 17609270
- PMCID: PMC1951452
- DOI: 10.1128/JVI.00647-07
Immunogenicity of influenza virus vaccine is increased by anti-gal-mediated targeting to antigen-presenting cells
Abstract
This study describes a method for increasing the immunogenicity of influenza virus vaccines by exploiting the natural anti-Gal antibody to effectively target vaccines to antigen-presenting cells (APC). This method is based on enzymatic engineering of carbohydrate chains on virus envelope hemagglutinin to carry the alpha-Gal epitope (Gal alpha 1-3Gal beta 1-4GlcNAc-R). This epitope interacts with anti-Gal, the most abundant antibody in humans (1% of immunoglobulins). Influenza virus vaccine expressing alpha-Gal epitopes is opsonized in situ by anti-Gal immunoglobulin G. The Fc portion of opsonizing anti-Gal interacts with Fc gamma receptors on APC and induces effective uptake of the vaccine virus by APC. APC internalizes the opsonized virus to transport it to draining lymph nodes for stimulation of influenza virus-specific T cells, thereby eliciting a protective immune response. The efficacy of such an influenza vaccine was demonstrated in alpha 1,3galactosyltransferase (alpha 1,3GT) knockout mice, which produce anti-Gal, using the influenza virus strain A/Puerto Rico/8/34-H1N1 (PR8). Synthesis of alpha-Gal epitopes on carbohydrate chains of PR8 virus (PR8(alpha gal)) was catalyzed by recombinant alpha1,3GT, the glycosylation enzyme that synthesizes alpha-Gal epitopes in cells of nonprimate mammals. Mice immunized with PR8(alpha gal) displayed much higher numbers of PR8-specific CD8(+) and CD4(+) T cells (determined by intracellular cytokine staining and enzyme-linked immunospot assay) and produced anti-PR8 antibodies with much higher titers than mice immunized with PR8 lacking alpha-Gal epitopes. Mice immunized with PR8(alpha gal) also displayed a much higher level of protection than PR8 immunized mice after being challenged with lethal doses of live PR8 virus. We suggest that a similar method for increasing immunogenicity may be applicable to avian influenza vaccines.
Figures
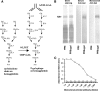
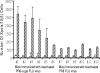
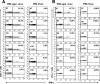
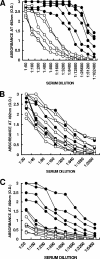
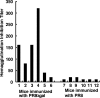
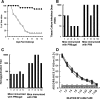
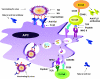
Similar articles
-
Increased immunogenicity of tumor vaccines complexed with anti-Gal: studies in knockout mice for alpha1,3galactosyltransferase.Cancer Res. 1999 Jul 15;59(14):3417-23. Cancer Res. 1999. PMID: 10416604
-
Increased immunogenicity of human immunodeficiency virus gp120 engineered to express Galalpha1-3Galbeta1-4GlcNAc-R epitopes.J Virol. 2006 Jul;80(14):6943-51. doi: 10.1128/JVI.00310-06. J Virol. 2006. PMID: 16809300 Free PMC article.
-
Amplifying immunogenicity of prospective Covid-19 vaccines by glycoengineering the coronavirus glycan-shield to present α-gal epitopes.Vaccine. 2020 Sep 29;38(42):6487-6499. doi: 10.1016/j.vaccine.2020.08.032. Epub 2020 Aug 19. Vaccine. 2020. PMID: 32907757 Free PMC article. Review.
-
Increasing Efficacy of Enveloped Whole-Virus Vaccines by In situ Immune-Complexing with the Natural Anti-Gal Antibody.Med Res Arch. 2021 Jul;9(7):2481. doi: 10.18103/mra.v9i7.2481. Epub 2021 Jul 10. Med Res Arch. 2021. PMID: 34853815 Free PMC article.
-
The alpha-gal epitope and the anti-Gal antibody in xenotransplantation and in cancer immunotherapy.Immunol Cell Biol. 2005 Dec;83(6):674-86. doi: 10.1111/j.1440-1711.2005.01366.x. Immunol Cell Biol. 2005. PMID: 16266320 Review.
Cited by
-
Biosynthesis of α-Gal Epitopes (Galα1-3Galβ1-4GlcNAc-R) and Their Unique Potential in Future α-Gal Therapies.Front Mol Biosci. 2021 Nov 4;8:746883. doi: 10.3389/fmolb.2021.746883. eCollection 2021. Front Mol Biosci. 2021. PMID: 34805272 Free PMC article. Review.
-
Augmenting Vaccine Immunogenicity through the Use of Natural Human Anti-rhamnose Antibodies.ACS Chem Biol. 2018 Aug 17;13(8):2130-2142. doi: 10.1021/acschembio.8b00312. Epub 2018 Jul 2. ACS Chem Biol. 2018. PMID: 29916701 Free PMC article.
-
Covalent decoration of adenovirus vector capsids with the carbohydrate epitope αGal does not improve vector immunogenicity, but allows to study the in vivo fate of adenovirus immunocomplexes.PLoS One. 2017 May 4;12(5):e0176852. doi: 10.1371/journal.pone.0176852. eCollection 2017. PLoS One. 2017. PMID: 28472163 Free PMC article.
-
Live unattenuated vaccines for controlling viral diseases, including COVID-19.J Med Virol. 2021 Apr;93(4):1943-1949. doi: 10.1002/jmv.26453. Epub 2020 Sep 29. J Med Virol. 2021. PMID: 32833258 Free PMC article. Review.
-
Factors Affecting Anti-Glycan IgG and IgM Repertoires in Human Serum.Sci Rep. 2016 Jan 19;6:19509. doi: 10.1038/srep19509. Sci Rep. 2016. PMID: 26781493 Free PMC article.
References
-
- Bottrel, R. L. A., W. O. Dutra, F. A. Martins, B. Gontijo, E. Carvalho, M. Barral-Netto, A. Barral, R. P. Almeida, W. Mayrink, R. Locksley, and K. J. Gollob. 2001. Flow cytometric determination of cellular sources and frequencies of key cytokine-producing lymphocytes directed against recombinant LACK and soluble Leishmania antigen in human cutaneous leishmaniasis. Infect. Immun. 69:3232-3239. - PMC - PubMed
-
- Celis, E., and T. W. Chang. 1984. Antibodies to hepatitis B surface antigen potentiate the response of human T lymphocyte clones to the same antigen. Science 224:297-299. - PubMed
-
- Chen, Z. C., M. Tanemura, and U. Galili. 2001. Synthesis of α-Gal epitopes (Galα1-3Galβ1-4GlcNAc-R) on human tumor cells by recombinant α1,3galactosyltransferase produced in Pichia pastoris. Glycobiology 11:577-586. - PubMed
Publication types
MeSH terms
Substances
Grants and funding
LinkOut - more resources
Full Text Sources
Other Literature Sources
Medical
Research Materials