The redox-switch domain of Hsp33 functions as dual stress sensor
- PMID: 17515905
- PMCID: PMC2782886
- DOI: 10.1038/nsmb1244
The redox-switch domain of Hsp33 functions as dual stress sensor
Abstract
The redox-regulated chaperone Hsp33 is specifically activated upon exposure of cells to peroxide stress at elevated temperatures. Here we show that Hsp33 harbors two interdependent stress-sensing regions located in the C-terminal redox-switch domain of Hsp33: a zinc center sensing peroxide stress conditions and an adjacent linker region responding to unfolding conditions. Neither of these sensors works sufficiently in the absence of the other, making the simultaneous presence of both stress conditions a necessary requirement for Hsp33's full activation. Upon activation, Hsp33's redox-switch domain adopts a natively unfolded conformation, thereby exposing hydrophobic surfaces in its N-terminal substrate-binding domain. The specific activation of Hsp33 by the oxidative unfolding of its redox-switch domain makes this chaperone optimally suited to quickly respond to oxidative stress conditions that lead to protein unfolding.
Conflict of interest statement
COMPETING INTERESTS STATEMENT
The authors declare no competing financial interests.
Figures
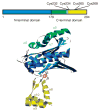
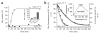
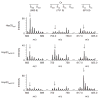
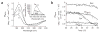
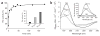
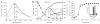
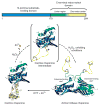
Similar articles
-
Activation of the redox-regulated molecular chaperone Hsp33--a two-step mechanism.Structure. 2001 May 9;9(5):377-87. doi: 10.1016/s0969-2126(01)00599-8. Structure. 2001. PMID: 11377198
-
Unfolding of metastable linker region is at the core of Hsp33 activation as a redox-regulated chaperone.J Biol Chem. 2010 Apr 9;285(15):11243-51. doi: 10.1074/jbc.M109.084350. Epub 2010 Feb 5. J Biol Chem. 2010. PMID: 20139072 Free PMC article.
-
A Role of Metastable Regions and Their Connectivity in the Inactivation of a Redox-Regulated Chaperone and Its Inter-Chaperone Crosstalk.Antioxid Redox Signal. 2017 Nov 20;27(15):1252-1267. doi: 10.1089/ars.2016.6900. Epub 2017 Apr 10. Antioxid Redox Signal. 2017. PMID: 28394178
-
Redox-regulated molecular chaperones.Cell Mol Life Sci. 2002 Oct;59(10):1624-31. doi: 10.1007/pl00012489. Cell Mol Life Sci. 2002. PMID: 12475172 Free PMC article. Review.
-
Oxidative stress: Protein folding with a novel redox switch.Curr Biol. 1999 Jun 3;9(11):R400-2. doi: 10.1016/s0960-9822(99)80253-x. Curr Biol. 1999. PMID: 10359689 Review.
Cited by
-
Order out of disorder: working cycle of an intrinsically unfolded chaperone.Cell. 2012 Mar 2;148(5):947-57. doi: 10.1016/j.cell.2012.01.045. Cell. 2012. PMID: 22385960 Free PMC article.
-
Thermodynamic analysis of a molecular chaperone binding to unfolded protein substrates.Biochemistry. 2010 Feb 16;49(6):1346-53. doi: 10.1021/bi902010t. Biochemistry. 2010. PMID: 20073505 Free PMC article.
-
Interplay of cellular cAMP levels, {sigma}S activity and oxidative stress resistance in Escherichia coli.Microbiology (Reading). 2009 May;155(Pt 5):1680-1689. doi: 10.1099/mic.0.026021-0. Epub 2009 Apr 16. Microbiology (Reading). 2009. PMID: 19372151 Free PMC article.
-
Redox regulation of the human dual specificity phosphatase YVH1 through disulfide bond formation.J Biol Chem. 2009 Aug 21;284(34):22853-64. doi: 10.1074/jbc.M109.038612. Epub 2009 Jun 30. J Biol Chem. 2009. PMID: 19567874 Free PMC article.
-
Conditionally and transiently disordered proteins: awakening cryptic disorder to regulate protein function.Chem Rev. 2014 Jul 9;114(13):6779-805. doi: 10.1021/cr400459c. Epub 2014 Feb 6. Chem Rev. 2014. PMID: 24502763 Free PMC article. Review. No abstract available.
References
-
- Imlay JA. Pathways of oxidative damage. Annu Rev Microbiol. 2003;57:395–418. - PubMed
-
- Holmgren A. Antioxidant function of thioredoxin and glutaredoxin systems. Antioxid Redox Signal. 2000;2:811–820. - PubMed
-
- Sies H. Oxidative stress: from basic research to clinical application. Am J Med. 1991;91:31S–38S. - PubMed
-
- Paget MS, Buttner MJ. Thiol-based regulatory switches. Annu Rev Genet. 2003;37:91–121. - PubMed
Publication types
MeSH terms
Substances
Grants and funding
LinkOut - more resources
Full Text Sources
Molecular Biology Databases