Biogenesis and function of multivesicular bodies
- PMID: 17506697
- PMCID: PMC2911632
- DOI: 10.1146/annurev.cellbio.23.090506.123319
Biogenesis and function of multivesicular bodies
Abstract
The two major cellular sites for membrane protein degradation are the proteasome and the lysosome. Ubiquitin attachment is a sorting signal for both degradation routes. For lysosomal degradation, ubiquitination triggers the sorting of cargo proteins into the lumen of late endosomal multivesicular bodies (MVBs)/endosomes. MVB formation occurs when a portion of the limiting membrane of an endosome invaginates and buds into its own lumen. Intralumenal vesicles are degraded when MVBs fuse to lysosomes. The proper delivery of proteins to the MVB interior relies on specific ubiquitination of cargo, recognition and sorting of ubiquitinated cargo to endosomal subdomains, and the formation and scission of cargo-filled intralumenal vesicles. Over the past five years, a number of proteins that may directly participate in these aspects of MVB function and biogenesis have been identified. However, major questions remain as to exactly what these proteins do at the molecular level and how they may accomplish these tasks.
Figures
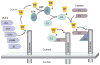
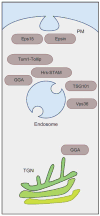
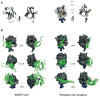
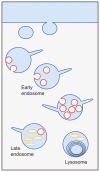
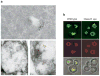
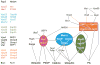
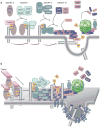
Similar articles
-
Vps27-Hse1 and ESCRT-I complexes cooperate to increase efficiency of sorting ubiquitinated proteins at the endosome.J Cell Biol. 2003 Oct 27;163(2):237-43. doi: 10.1083/jcb.200305007. J Cell Biol. 2003. PMID: 14581452 Free PMC article.
-
A family of tetraspans organizes cargo for sorting into multivesicular bodies.Dev Cell. 2015 May 4;33(3):328-42. doi: 10.1016/j.devcel.2015.03.007. Dev Cell. 2015. PMID: 25942624 Free PMC article.
-
Cell-free reconstitution of multivesicular body (MVB) cargo sorting.Methods Mol Biol. 2015;1270:115-24. doi: 10.1007/978-1-4939-2309-0_9. Methods Mol Biol. 2015. PMID: 25702113
-
Ubiquitin and endocytic protein sorting.Essays Biochem. 2005;41:81-98. doi: 10.1042/EB0410081. Essays Biochem. 2005. PMID: 16250899 Review.
-
ESCRT and Membrane Protein Ubiquitination.Prog Mol Subcell Biol. 2018;57:107-135. doi: 10.1007/978-3-319-96704-2_4. Prog Mol Subcell Biol. 2018. PMID: 30097773 Review.
Cited by
-
Exosomal Long Non-coding RNAs: Emerging Players in the Tumor Microenvironment.Mol Ther Nucleic Acids. 2020 Oct 4;23:1371-1383. doi: 10.1016/j.omtn.2020.09.039. eCollection 2021 Mar 5. Mol Ther Nucleic Acids. 2020. PMID: 33738133 Free PMC article. Review.
-
Rab7 silencing prevents μ-opioid receptor lysosomal targeting and rescues opioid responsiveness to strengthen diabetic neuropathic pain therapy.Diabetes. 2013 Apr;62(4):1308-19. doi: 10.2337/db12-0590. Epub 2012 Dec 10. Diabetes. 2013. PMID: 23230081 Free PMC article.
-
The emerging roles of exosomal circRNAs in diseases.Clin Transl Oncol. 2021 Jun;23(6):1020-1033. doi: 10.1007/s12094-020-02485-6. Epub 2020 Sep 15. Clin Transl Oncol. 2021. PMID: 32935262 Free PMC article. Review.
-
The importance of claudin-7 palmitoylation on membrane subdomain localization and metastasis-promoting activities.Cell Commun Signal. 2015 Jun 9;13:29. doi: 10.1186/s12964-015-0105-y. Cell Commun Signal. 2015. PMID: 26054340 Free PMC article.
-
Gold nanoparticles (AuNPs) impair LPS-driven immune responses by promoting a tolerogenic-like dendritic cell phenotype with altered endosomal structures.Nanoscale. 2021 Apr 30;13(16):7648-7666. doi: 10.1039/d0nr09153g. Nanoscale. 2021. PMID: 33928963 Free PMC article.
References
-
- Alam SL, Langelier C, Whitby FG, Koirala S, Robinson H, et al. Structural basis for ubiquitin recognition by the human ESCRT-II EAP45 GLUE domain. Nat Struct Mol Biol. 2006;13:1029–30. - PubMed
Publication types
MeSH terms
Substances
Grants and funding
LinkOut - more resources
Full Text Sources
Miscellaneous